Chimeric antigen receptor T cells for the treatment of lymphoma
Introduction
Allogeneic hematopoietic stem cell transplantation (HSCT) was the first example of cellular immune therapy to treat cancer. Initially, HSCT was used to restore hematopoiesis following high dose chemotherapy and radiation given to eradicate cancer cells (1). However, it was soon discovered that the allogeneic immune system has anti-tumor activity, now termed “graft-versus-leukemia” (GVL) (2,3), facilitating the development of reduced-intensity conditioning regimens that are more reliant on GVL for therapeutic benefit. In circumstances where sufficient GVL cannot be achieved by discontinuation of immunosuppression, donor leukocyte infusion (DLI) may be effective at enhancing GVL and providing disease control. This observation, along with the observation of increased relapse following T-cell depleted HSCT, confirmed the importance of T lymphocytes in tumor cell eradication by GVL. While SCT can be potentially curative, high incidence of morbidity and mortality compromises outcomes and limits feasibility of this treatment in patients with comorbidities. In addition, cancer cells can escape immunosurveillance mediated by allogeneic T cells, thereby leading to disease relapses. Therefore, a highly potent, engineered cell therapy that is specifically designed to target cancer cells without replacing the entire marrow has the potential to substantially improve the effectiveness of immune based therapy for lymphoma.
T cells are attractive as anti-cancer agents for several reasons including potent activity against tumor cells, robust potential for in vivo self-expansion, antigen-specificity, and the ability to generate immune memory providing the possibility for long-term tumor surveillance. Following many years of research, various adoptive T-cell therapies have been employed in the treatment of cancer. In addition to DLI, ex vivo activated tumor infiltrating lymphocytes (TILs) and T cells from blood expanded ex vivo against tumor-associated antigens have all been used therapeutically to varying degrees of success. All of these approaches rely on the natural T cell repertoire provided by the endogenous T cell receptor (TCR) for activity. Advances in genetic engineering have enabled the efficient transfer of genes encoding TCRs recognizing selected target antigens from processed cell surface or intracellular proteins into T cells. This results in redirected specificity and potent anti-tumor activity (4-6). However, TCR-based gene modified adoptive therapy is major histocompatibility complex (MHC) restricted thus limited to a subset of patients expressing a specific MHC type (7). An alternative type of antigen specific receptor that has proven to be extremely effective in clinical trials is the chimeric antigen receptor (CAR) (8,9). We will review the structure of CAR, the results of landmark clinical trials utilizing CAR T cells for the treatment of lymphoma, toxicities of CAR T cell therapy, the causes of failure of CAR T cell therapy, and new developments to overcome those limitations.
Introduction to CARs
CARs are artificial constructs that, when introduced into T cells, enable recognition and killing of target cells in highly specific and MHC independent manner. This is accomplished through an antigen recognition motif that is typically derived from a target-specific antibody that is fused to an intracellular CD3 signaling domain (CD3ζ), termed “first-generation” CAR. Incorporation of a T-cell costimulatory (e.g., CD28, 4-1BB) domain was shown to dramatically increase the potency of CAR T cells such that the majority of clinical trials use these “second-generation” constructs (10-12). “Third-generation” CARs that incorporate more than one costimulatory domain have been tested but it remains unclear as to whether these constructs provide advantages over second-generation constructs (Figure 1). The engineered nature of CARs allows for a high degree of flexibility and provides opportunities for further modifications to control CAR T cell behavior (7,11,13).
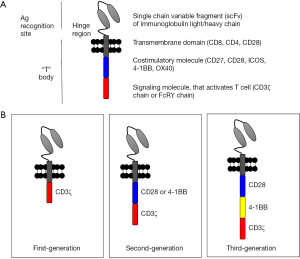
The extracellular domain of CAR consists of the antigen recognition motif, usually in the form of a single chain variable fragment (scFv) derived from an antibody. In addition, many CARs also contain an extracellular hinge/linker region that provides some distance from the cell membrane to the antigen-binding region. Studies suggest the immunological synapse distance formed between the CAR and the target antigen may impact CAR functionality (14-16). For example, maximal efficacy of CD22 CAR was only obtained when CD22 was modified so that the target epitope was positioned in close proximity to the cell membrane (17). Although scFv binding domains are most frequently used in CAR constructs, in theory, any motif that binds to a tumor target may be incorporated into CARs (18). It is likely that there will be other CAR constructs developed in the future that use domains other than scFv, given the challenges associated with scFv folding.
The intracellular domain of “first-generation” CARs contains only the CD3ζ signaling domain. Considering the role of costimulatory molecules in T cell activation, several groups developed second-generation of CARs that incorporates the co-signaling motifs derived from CD28 or 4-1BB intrinsically in the CAR construct, thereby ensuring that both signal 1 (CD3ζ signaling) and signal 2 (costimulatory signaling) are available to fully activate the CAR T cell (19,20). Subsequently, a number of other signaling motifs such as ICOS (21,22) and CD27 (23,24) have been tested. Both CD28 and 4-1BB costimulation induce IL-2 production by T cells (25-27), enhance survival of activated T cells (28,29), and support cytotoxic activity of T cells (30-33). Depending on how the CAR is designed, the engineered CAR T cells take on the phenotype of the T cells for which it is engineered. For example, embedding CD28 costimulatory domain into the CAR was shown to make CD8+ CAR T cells behave like effector memory T cells, while CD8+ CAR T cells with 4-1BB signaling motif in the CAR behaves like central memory T cells (34). When CAR T cells are engineered with ICOS signaling domain, the CAR T cells have a Th1/Th17 T cell phenotype (22). When regulatory T (Treg) cells were used to generate CAR T cells, these cells maintained Treg functions (35). Constructing third-generation CAR to include both CD28 and 4-1BB signaling domains in tandem seems to provide an additive effect on CAR T cell proliferation (12), with predominant number of CAR T-cells being CD8+ effector memory T cells (12). Therefore, CAR T cells may be engineered to take on the desired T cell phenotype (22,35,36).
CAR T cell potency may be enhanced by expanding CAR T cell specificity and/or by enhancing antigenic stimulation. In fact, CAR T cells require certain threshold of interaction for the target antigen for activation (37). One method for improving CAR T cell specificity is to target multiple tumor associated antigens simultaneously, as demonstrated by bi-specific and/or tandem CARs (38,39). The tandem CAR T cells also showed less expression of exhaustion markers PD-1, LAG3, and TIM3 when compared to other CAR T cells (40).
Combination therapy involving CAR T cells may also be used to either increase the tumor antigen expression of the tumor, enhance CAR T cell survival and persistence, or inhibit CAR T cell inhibitors. Poor antigenic stimuli may be prevailed by either amplifying the signal transduction cascade (for example, costimulatory molecules) or by enhancing synapse formation. Kuramitsu et al. demonstrated that lenalidomide enhances CAR T cell toxicity by enhancing CAR-antigen immunological synapse (41). Otahal et al. showed that lenalidomide increased IFNγ production upon antigenic stimulation in CAR T cells in a dose-dependent manner (42). CAR T cell survival and persistence appears to be essential for prolonged anti-tumor activity. T cell growth factors, such as IL-2, IL-7, and IL-15, etc., may be co-infused/co-expressed with CAR T cells to enhance CAR T cell survival and persistence (43). Hurton et al. demonstrated co-expressing membrane bound form of IL-15 aids CAR T cell persistence in addition to promoting memory stem cell phenotype (44). Co-administering CAR T cells with ibrutinib has been explored and may be a highly effective treatment that targets both surface antigen (CD19) as well as intracellular (BTK signaling) inhibition of tumor survival and growth (45). Checkpoint inhibitors, such as PD-1/PD-L1 interactions, are upregulated following antigenic stimulation on T cells and function to prevent overt T cell response (46,47). Clinical studies are underway to see if co-administering checkpoint inhibitor antagonists with CAR T-cells may prolong CAR T-cell persistence, and survival (Table 1).
Table 1
NCT # | Center | Target | Phase | Lymphoma types | Titles | Sponsors |
---|---|---|---|---|---|---|
NCT01853631 | Houston Methodist Hospital Texas Children’s Hospital | CD19 | Phase I | NHL | Activated T-Cells Expressing 2nd or 3rd Generation CD19-Specific CAR, Advanced B-Cell NHL, ALL, and CLL (SAGAN) | Baylor College of Medicine |
NCT02706405 | Fred Hutch/University of Washington Cancer Consortium | CD19 | Phase I | DLBCL, PMBCL | JCAR014 and Durvalumab in Treating Patients With Relapsed or Refractory B-cell Non-Hodgkin Lymphoma | Fred Hutchinson Cancer Research Center |
NCT02926833 | Multicenter | CD19 | Phase I/II | DLBCL | A Study Evaluating KTE-C19 in Combination with Atezolizumab in Subjects With Refractory Diffuse Large B-Cell Lymphoma (DLBCL) | Kite Pharma, Inc. |
NCT02650999 | University of Philadelphia, Philadelphia, PA | CD19 | Phase I/II | DLBCL, FL | Phase I/II Study of Pembrolizumab in Patients Failing to Respond to or Relapsing After Anti-CD19 Chimeric Antigen Receptor Modified T Cell Therapy for Relapsed or Refractory CD19+ Lymphomas | Abramson Cancer Center of University of Philadelphia |
NCT02659943 | National Institutes of Health Clinical Center, | CD19 | Phase I | B-cell lymphoma | T Cells Expressing a Fully-human AntiCD19 Chimeric Antigen Receptor for Treating B-cell Malignancies | National Cancer Institute |
NCT03097770 | Chinese PLA General Hospital, Beijing, China | CD19/CD20 | Unknown | DLBCL, FL, MCL | Treatment of Relapsed and/or Chemotherapy Refractory B-cell Malignancy by Tandem CAR T Cells Targeting CD19 and CD20 | Chinese PLA General Hospital |
NCT03185494 | Chinese PLA General Hospital, Beijing, China | CD19/CD22 | Phase I/II | DLBCL, FL, MCL | Treatment of Relapsed and/or Chemotherapy Refractory B-cell Malignancy by Tandem CAR T Cells Targeting CD19 and CD22 | Chinese PLA General Hospital |
NCT01735604 | Chinese PLA General Hospital, Beijing, China | CD20 | Phase I/II | DLBCL, FL, MCL | Genetically Engineered Lymphocyte Therapy in Treating Patients With Lymphoma That is Resistant or Refractory to Chemotherapy | Chinese PLA General Hospital |
NCT02650414 | Children’s Hospital of Pennsylvania | CD22 | Phase I | B-cell lymphomas | CD22 Redirected Autologous T Cells for ALL | University of Pennsylvania |
NCT01316146 | Multicenter | CD30 | Phase I | HL, NHL | Administration of T Lymphocytes for Hodgkin’s Lymphoma and Non-Hodgkin’s Lymphoma (CART CD30) | UNC Lineberger Comprehensive Cancer Center |
NCT03049449 | National Institutes of Health | CD30 | Phase I | ALCL, HL, enteropathy-associated TCL, extranodal NK T-cell lymphoma | T Cells Expressing a Fully-Human Anti-CD30 Chimeric Antigen Receptor for Treating CD30-Expressing Lymphomas | National Cancer Institute |
NCT00881920 | Houston Methodist Hospital Texas Children’s Hospital | Kappa Immunoglobulin | Phase I | Lymphoma | Kappa-CD28 T Lymphocytes, Chronic Lymphocytic Leukemia, B-cell Lymphoma or Multiple Myeloma, CHARKALL | Baylor College of Medicine |
NCT02706392 | Fred Hutch/University of Washington Cancer Consortium | ROR1R | Phase I | MCL | Genetically Modified T-Cell Therapy in Treating Patients With Advanced ROR1+ Malignancies | Fred Hutchinson Cancer Research Center |
HL, Hodgkin lymphoma; NHL, non-HL; DLBCL, diffuse large B-cell lymphoma; PMBCL, primary mediastinal B-cell lymphoma; FL, follicular lymphoma; MCL, mantle cell lymphoma; ALCL, anaplastic large cell lymphoma; TCL, T-cell lymphoma.
Clinical trials utilizing CAR T cells in the treatment of lymphoma
There are an estimated 72,580 new cases of non-Hodgkin lymphoma (NHL) diagnosed every year, with an estimated 20,150 deaths (SEER database, 2016). Up to 85% of NHL is B-cell lymphoma, including diffuse large B-cell lymphoma (DLBCL), follicular lymphoma (FL), primary mediastinal B-cell lymphoma (PMBCL), and mantle cell lymphoma (MCL). With conventional immune-chemotherapy, many patients with NHL are cured. However, despite the numerous drugs and combinations available, one third of patients eventually relapse due to incomplete eradication of tumor cells (48). With current treatments, relapsed and/or refractory (R/R) aggressive B-cell NHL has poor outcomes (49). Allogeneic SCT is a treatment option for relapsed/refractory patients, however, it carries high treatment-related mortality, and many patients are not able to tolerate it (50-52). The salvage therapy for R/R NHL is not effective and requires development of new therapies.
The success of CAR T-cell therapy has been demonstrated with CD19-directed CAR T cells, namely with pediatric and adult acute lymphoblastic leukemia (ALL), with complete remission (CR) of near 90% (53) and led to the FDA approving CTL019 (Novartis, Basel, Switzerland) for treatment of pediatric B-ALL. Although CD19-directed CAR T cell therapy has less impressive effect on slower growing CD19 positive B-cell malignancies such as NHL, CAR T cells may offer a better prognosis for relapsed patient with NHL than chemotherapy. In addition to CD19, there are growing numbers of targets for NHL and HL that have been validated with Ab-based therapy, and are being translated as targets for CAR-based therapy for patients with lymphomas.
Many clinical trials utilizing CAR T cells demonstrated the critical importance of lymphocyte-depletion prior to cell infusion (7,54-57), and showed that lymphocyte-depletion enhanced in vivo persistence of CAR T cells. The increased proliferation and persistence of adoptively transferred T cells may involve the elevation of serum cytokines such as IL-15 and IL-7 resulting from lymphodepletion (58).
CAR T cells targeting CD19 for B-cell lymphoma
The results of selected published clinical trials utilizing CD19 CAR T cells for patients with B-cell lymphoma are summarized in Table 2. In 2010, a clinical trial demonstrated significant efficacy of CD19 CAR T cell therapy in lymphoma. A patient with advanced FL received 2 doses (1×108 on day 1, and 3×108 cells with IL-2 on day 2) of autologous CD19/CD28 CAR T cells after lymphodepletion with cyclophosphamide and fludarabine. This patient previously received multiple lines of chemo- and immune-therapy (EPOCH-R, PACE, idiotype vaccine, ipilimumab) without response. With CD19 CAR T cell treatment, the lymphoma regressed and the patient achieved partial remission (54). B-cell aplasia lasted 32 weeks. Based on this encouraging result, additional patients with NHL were enrolled on this protocol (NCT00924326). Interim analysis was reported in 2012 demonstrating the efficacy of CD19/CD28 CAR T cells in indolent lymphomas: chronic lymphocytic leukemia (CLL) (n=4), FL (n=4), and splenic marginal zone lymphoma (MZL) (n=1). Six of 8 enrolled patients achieved objective response (three of four with CLL; 2 of 3 with FL) lasting 7–18+ months (59). Subsequent analysis reported in 2015 showed 15 more patients with B-cell malignancies who were treated with CD19 CAR T cells after failing 1–12 previous lines of therapy: 9 patients with DLBCL, 2 patients with indolent lymphomas, and 4 patients with CLL. At the time of publication, out of 13 evaluable patients, 12 (92%) achieved an objective clinical response, with 8 (62%) achieving complete response durable to >23 months (60). Most recently, Kochenderfer et al. reported results of additional 22 patients: DLBCL (n=19), FL (n=2), and MCL (n=1), who relapsed after undergoing 1–7 lines of therapy, including allogeneic SCT. Overall response rate (ORR) was 73%, with 55% CR, and 18% of partial response (PR), lasting 1–18+ months (61).
Table 2
References | Lymphoma types [patient number] | CAR T cells | Response rate [%] |
---|---|---|---|
Kochenderfer et al. 2010 | FL [1] | Autologous CD19/CD28 | ORR, 1/1 [100]; CRR, 0/1 [0] |
Kochenderfer et al. 2012 | FL [4], MZL [1] | Autologous CD19/CD28 | ORR, 4/4 [100]; CRR, 0/4 [0] |
Kochenderfer et al. 2015 | DLBCL [9], indolent NHL [1], MZL [1] | Autologous CD19/CD28 | ORR, 8/9 [89]; CRR, 5/9 [56] |
Kochenderfer et al. 2017 | DLBCL [19], TFL [4], FL [2], PMBCL [2], MCL [1] | Autologous CD19/CD28 | ORR, 16/22 [73]; CRR, 12/22 [55] |
Locke et al. 2017 (ZUMA-1) | DLBCL [7] | Autologous CD19/CD28 | ORR, 5/7 [71]; CRR, 4/7 [57] |
Wang et al. 2016 (NHL-1) | DLBCL [7], MCL [1] | Autologous CD19 first-generation (Tcm) | ORR, 7/8 [88]; CR, 5/8 [63] |
Wang et al. 2016 (NHL-2) | DLBCL [4], MCL [4] | Autologous CD19/CD28 (CD4/CD8 Tcm) | ORR, 8/8 [100]; CR, 8/8 [100] |
Turtle et al. 2016 | DLBCL [22], MCL [4], FL [6] | Autologous CD19/CD4-1BB | ORR, 19/30 [63]; CR, 10/30 [33] (two patients unevaluable) |
Schuster et al. 2015 | DLBCL [15], FL [13], MCL [2] | Autologous CD19/CD4-1BB | ORR, 16/30 [53]; CR, 13/30 [43] |
Abramson et al. 2016 (TRANSEND) | DLBCL [11], MCL [1] | Autologous CD19/4-1BB (1:1 CD4, CD8) | ORR, 9/12 [75]; CR, 8/12 [67] |
CAR, chimeric antigen receptor; MZL, marginal zone lymphoma; DLBCL, diffuse large B-cell lymphoma; NHL, non-Hodgkin lymphoma; PMBCL, primary mediastinal B-cell lymphoma; FL, follicular lymphoma; TFL, transformed FL; MCL, mantle cell lymphoma; Tcm, central memory enriched T cells; ORR, overall response rate; CRR, complete response rate; CR, complete remission.
Same CAR construct was used by Kite Pharma in a phase I (ZUMA-1) multicenter study (NCT02348216). In this study, seven patients with refractory DLBCL were treated with CD19/CD28 CAR T cells after receiving cyclophosphamide and fludarabine for lymphodepletion. Overall response was seen in 5 (71%) patients, and 4 patients achieved CR (57%) for up to 12+ months (62).
Wang et al. reported the results from two phase I clinical trials (NCT 01318317, NCT01815749) in lymphoma, coined NHL1 and NHL2, respectively (63). NHL1 study was designed to utilize autologous central memory enriched T cells (Tcm) to generate CD19 CAR T cells while NHL2 study was designed to utilize CD4/C8 selected Tcm cells to generate CD19 CAR T cells. In both studies, patients with relapsed/refractory NHL received CD19 CAR T cells 2 days following autologous SCT. In the NHL1 trial, eight patients (7 with DLBCL, and 1 with MCL) were treated with CD19 CAR T cells. Four of 8 patients were progression free for 2 years. In the NHL2 trial, 6 out of 8 patients (4 with DLBCL, and 4 with MCL) were progression free at 1 year (63).
Turtle et al. combined CD4+ CD19 CAR T cells with CD8+ CD19 CAR T cells at 1:1 ratio to treat patients with NHL (NCT01865617). Total of 32 patients were enrolled who received median 5 lines of therapy including autologous and allogeneic SCT (11 de novo DLBCL, 11 DLBCL transformed from FL, 4 MCL, 6 FL). Twelve patients were lymphodepleted with cyclophosphamide alone prior to CAR T cells and achieved 50% ORR and 8% CR. After modifying the lymphodepleting regimen by combining cyclophosphamide with fludarabine, additional 20 patients were enrolled with ORR 72%, and 50% CR (64). Overall, among 30 evaluable patients, 19 (63%) had ORR, and 10 (33%) achieved CR.
Phase II trial with CTL019 in heavily pretreated, relapsed/refractory NHL patients (15 DLBCL, 13 FL, and 2 MCL) is ongoing at University of Pennsylvania. The interim analysis showed ORR of 47% in DLBCL (6 CR, 1 PR), 73% in FL (7 CR, 1 PR), and 50% in MCL (65).
Abramson et al. reported (ASH 2016) their result of phase I study of CD19 CAR T cell (JCAR017) in relapsed/refractory NHL (11 DLBCL and 1 MCL were treated). Median number of previous therapy was 5, including auto and allogeneic SCT. In DLBCL group 9/11 (82%) OR, 8/11 (73%) CR, 1/11 (9%) PR, and 2/11 (18%) progressive disease (PD) were seen at the time of assessment. The MCL patient had PD at day 29 (66).
Autologous T cell engineering has been the most heavily investigated source of T cells in clinical trials. However, donor-derived CAR T cell is an attractive modality to generate “off shelf” product to avoid CART cell production failure, wait time for patient and, as such, is being actively investigated. Kochenderfer et al. reported utilization of donor-derived engineered CD19/CD28 CAR T cells in patients with various B-cell malignancies who had undergone previous allogeneic SCT (67). Lymphocytes were collected from the donors; CD19/CD28 CAR T cells were generated and infused in 10 patients with DLBCL (n=2), CLL (n=4), or MCL (n=4). Eight of 10 patients had overall response, 2 with PR, and 6 with stable disease (SD). In patients who relapsed after allogeneic SCT, DLI is a common practice to induce GVL for disease control (68); however, approximately one third of the patients develop graft vs. host disease (GVHD). Remarkably, none of the patients treated with donor-derived CAR T cells experience GVHD. In subsequent report, consisting of 20 total patients treated with donor-derived CD19/CD28 CAR T cells [NHL (n=10), CLL (n=5), ALL (n=5)], 8 (40%) patients demonstrated an objective response, with 6 (30%) patients achieving CR. Again, no patient experienced new acute GVHD after CAR T cell infusion (69). Although the potential benefit of using allogeneic CAR T cells is great, given the presence of native TCR in CAR T cells, the possibility of GVHD is still present (70). Certainly, targeted insertion of the CAR construct into the TCR loci would likely prevent GVHD (71); however, allogeneic CAR T cells may not be as potent due to the potential host vs. therapy mediated elimination of the allogeneic CAR T cells. While early studies with allogeneic CAR T cells show great promise, extensive evaluation and fine-tuning is warranted in the application of donor-derived/allogeneic CAR T cells. The results of published trials are summarized in Table 2.
CAR T cells targeting other antigens
CD20 is an attractive target due to the availability of effective monoclonal antibody rituximab that is widely used in clinical practice. Jensen et al. reported in 2010 the results of two patients with DLBCL treated with first-generation CD20 CAR T cells (72). Patients did not have any adverse event attributable to CD20 CAR T-cells. CD20 CAR T cells were administered following autologous SCT. Nevertheless, both patients achieved CR (72). Subsequently, Till et al. reported results of four patients (3 MCL, 1 FL) who received third-generation CD20 CAR T cells that consist of both CD28 and 4-1BB costimulatory molecules. One patient withdrew from the study. Two patients without evaluable diseases remained progression free at 12 and 24 months at the time of publication. One patient had PR, however relapsed at 12 months after CD20 CAR T cell infusion (73). Another phase I clinical trial treated 16 patients with NHL [7 NHL: 4 DLBCL, 2 lymphoplasmacytic lymphoma (LPL), 1 MCL; 2 CLL; 7 MM] with κCAR T cells that target immunoglobulin κ chain expressing B-lymphomas. Two patients with DLBCL achieved CR, two patients with LPL achieved PR, one patient with CLL had no response, one had SD for 6 weeks. Of seven patients with MM, 4 had stable disease lasting 2–17 month (74).
Targeting CD30 to treat HL and anaplastic large cell lymphoma (ALCL) has shown success with drug conjugated monoclonal antibodies, brentuximab vedotin. Utilizing CD30 CAR T cells is an attractive approach to avoid side effects of vedotin, and increase duration of response. Ramos et al. reported results of phase I study, utilizing second-generation CD30 CAR T cells. Nine patients with R/R HL [7], or ALCL [2] were treated with CD30 CAR T cells, 5 patients with HL had overall response (2 CR lasting 2.5 years, and 2 years; 3 transient SD), and 1 patient with ALCL achieved CR for 9 months (75).
There are over 200 clinical trials registered at www.clincaltrials.gov as of September 2017, utilizing CAR T cell therapy for lymphoma, and targeting various antigens that express on lymphoma. Table 1 summarizes select ongoing phase I/II studies utilizing novel CAR T cells targeting new antigens and/or used in combination with immunomodulatory drugs that are currently enrolling patients.
Toxicity of CAR T cell therapy
The remarkable response rate to the treatment with CAR T cell therapy comes with substantial toxicities. These toxicities are attributable to the lymphodepleting regimen, potential side effect of utilizing retro-viral transduction for genetic manipulation, as well as toxicities specifically attributable to CAR T cells.
Toxicities resulting from lymphodepleting agents such as myelosuppression, nausea, vomiting, diarrhea, myalgia, and fatigue are expected to be transient and are easily managed with appropriate supportive care. Tumor lysis syndrome (TLS) following lymphodepletion and CAR T cell infusion is well-established complication, especially with bulky disease. TLS may be managed with appropriate supportive care. Potential side effects of gene therapy include insertional mutagenesis and the emergence of replication-competent retrovirus (RCR). Insertional mutagenesis is theoretically possible due to the promiscuity of retroviral vectors, and it has been observed, e.g., in the setting of infants treated for X-linked severe combined immunodeficiency (X-SCID) using retroviral vector-mediated gene transfer into CD34+ bone marrow stem cells. Genetic manipulation using retroviral vectors to transduce mature T cells seems to be a safe procedure and will require continued follow-up for confirmation. Additionally, RCR emergence has not been observed with CAR T cell therapy to date. Another expected side effect of CD19 CAR T cell therapy is the elimination of B-cells, so called “on target off tumor effect”. B-cell aplasia sometimes serves to indicate CD19 CAR T cells are expanding/persisting and may be easily managed with monthly intravenous immunoglobulin (IVIG) infusions.
Cytokine storm and neurotoxicity are complications that are unique for immunotherapy, particularly CAR T cell therapy. The cytokine release syndrome (CRS) is defined clinically by group of symptoms including, but not limited to fever, muscle pain, nausea, diarrhea, headache, tachycardia, hypotension, rash, shortness of breath, coagulopathy, hemorrhage, thrombosis, elevated liver enzyme, pancytopenia that can lead to multi-organ failure requiring admission to the intensive care unit with vasopressor support, and intubation for respiratory failure. It is believed to be caused by the release of cytokines from cells following intense immune activation. A rapid rise in serum cytokine levels (cytokine storm) has been associated with systemic inflammatory response syndrome (SIRS), potentially leading to multi-organ dysfunction (MOD) (76,77). Early studies with CAR T cell therapy reported elevation of inflammatory cytokines, including IL-6, IFN-γ, IL-2, IL-7, and TNF (76). Targeting these cytokines, particularly IL-6, was shown to prevent/treat potentially fatal CRS (77). The rate and degree of CRS varies between clinical trials, with all grade CRS approaching 90% (76,77). Comparison is challenging due to differences in constructs used by various clinical trials, differences in dosing of the cells, differences in lymphodepleting regimens, and differences in grading system used to grade CRS.
Many clinical trials, particularity with CD19 CAR T cells have reported transient neurologic symptoms including cognitive impairment, confusion, visual hallucinations, lack of responsiveness to commands, ataxia, aphasia, tremors, seizures, and cerebral edema. Although the rate of neurotoxicities varies from 0% to over 50% (76), there were reports of grade 5 neurotoxicities in B-ALL, as well as in NHL patients. The exact mechanism of neurotoxicity is not fully understood. It has been reported that central nervous system (CNS) does not express CD19 antigen (78), and appears that neurotoxicity happens even in the absence of disease in CNS. Neurotoxicity after CAR T cells could occur with CRS as well as without. It is possible that T cells actively traffic to CNS, or cytokine produced by activated immune cells diffuse to CNS. More studies are required to understand the mechanism, prevention and treatment of neurotoxicity.
In the multicenter ZUMA-1 study utilizing CD19/CD28 CAR T cells for DLBCL, transformed FL (TFL), PMBCL, grade ≥3 CRS and neurotoxicity occurred in 13% and 28%, respectively, among 101 patients who received CAR T cells (79). In the JULIET study utilizing CD19/4-1BB CAR T cells for R/R DLBCL, among 51 patients, CRS occurred in 57% of the patients, with grade ≥3 CRS occurring in 26% of the patients, 16% received tocilizumab for the management of CRS, 13% of patients developed grade ≥3 neurotoxicity (80). In TRANSCEND study utilizing CD19/4-1BB CAR T cells, 28 patients were treated and evaluated, 35% patients developed grade 1–2 CRS; however, none of them had grade ≥3 CRS. One patient received tocilizumab for management of CRS, 5 patients developed neurotoxicity (17%), 4 of them had grade ≥3 (14% patients). All events resolved. Generally, the onset of CRS and neurotoxicity were observed at 5–11 days, though it varied depending on construct utilized (81).
Early CRS and neurotoxicity intervention consists of steroids and/or the administration of anti-IL6R monoclonal antibody (mAb) therapy (tocilizumab). Interestingly, the severity and frequency of CRS is lower with lymphomas in comparison with leukemia; nevertheless, grade 3 or higher toxicity is reported in >60% of the patients. Together, while much of toxicities associated with CAR T cell therapy are manageable, finding ways to mitigate these toxicities without compromising efficacy would dramatically improve CAR T cell therapy.
Failure of CAR T cell therapy in lymphoma
While ongoing clinical studies demonstrate excellent response with CAR T cell therapy, lack of response or relapse are reported. The cause for ineffective CAR T cell therapy is multifactorial including immunomodulation by tumors, CAR T cell design, and tumor heterogeneity/plasticity (82). Cancer-immunosurveillance (83) is a crucial component of preventing cancer formation (84-86). The same mechanisms by which cancer cells escape immunosurveillance appear to play a role in suppressing/escaping CAR T cell therapy. For example, checkpoint inhibitors are known to suppress anti-cancer immune responses (87) and limit CAR T-cell efficacy (88,89). In addition, loss of target antigen has been well observed with CAR T cell therapy (82,90-93). Sotillo et al. (94) showed that under CD19 CAR T cell evolutionary pressure, leukemic cells develop mutations, undergo alternative splicing of CD19 gene, and is no longer recognized by CAR T cells. Jacoby et al. demonstrated that leukemic cells lose CD19 expression and develop resistance to CD19 CAR T cells (82). Lastly, an intriguing phenomenon was observed when in the presence of CAR T cells, leukemic cells were able to completely switch from lymphoid to myeloid lineage, demonstrating even greater plasticity of cancer cells than was original thought (82).
Lymphoma cells are uniquely more challenging targets for CAR T cells than leukemia cells. Leukemia cells circulate in the marrow and peripheral blood, therefore are easily accessible for CAR T cells. In contrast, CAR T cell have limited access to lymphoma due to physical barrier, limited blood vessels in solid tumor, as well as the down-regulation of integrins and selectins that are critical for T cell migration. In addition, tumor microenvironment inhibits CAR T cells by releasing immunosuppressive cytokines and ligands such as PD-L, TGF-B, and IL-10 (95).
Loss or down-regulation of CD19 antigenic target and lineage switching are well documented in ALL (82); however, this has not been observed in lymphoma to date. It is possible that as more patients with lymphoma undergo CD19 CAR T cell therapy, we will see CD19 antigenic loss as well. It is also possible that while lymphomas are terminally differentiated tumors, leukemia has greater plasticity due to the tumor rising from less committed population of cells. Lymphomas have aberrant cell survival signal transduction pathways, including Bcl2, Bcl6, BRAF, TP53, NRAS, PI3K/AKT/mTOR signaling, BTK signaling, LYN/SYK signaling, JAK2/STAT, and PKCβ signaling pathways (96,97). Relapsed/refractory NHL cells resistant to apoptotic signals presented by native immune cells, and cytotoxic drugs, in addition to activation of pro-survival signaling, could also make lymphoma more resistant to CAR T cell therapy. Identifying key mechanism(s) of lymphoma escape from CAR T cell treatment would contribute to designing more effective CAR T cells.
Conclusions
The advent of genetically modified immunotherapy has created much enthusiasm and hope for difficult to treat ailments. Several recent clinical trials have demonstrated success of engineered T cell therapies for the treatment of B cell malignancies. Although there are still challenges in the treatment of lymphoma, CAR T cells provide new hope for relapse/refractory lymphomas. The downside to the therapy is that patients who receive CAR T cells are at risk for serious and potentially life-threatening side effects such as CRS and neurotoxicity. Therefore, therapy should be administered in large centers that have experience and built-in infrastructure for CAR T cell treatment. CAR T cell therapy combines cell therapy, gene therapy, and immunotherapy features. The real-world challenges include logistics surrounding CAR T cell therapy, time for manufacturing of cells, and high cost of therapy. Promising results utilizing new targets, development of bispecific CARs, and introduction of combination therapy will improve the response rate, in addition to decreasing rate of relapse. One important question that needs to be addressed is the duration of response and the role of SCT after CAR T cell therapy, and whether CAR T cell therapy is a curative treatment option for lymphoma or a bridge to SCT. Many strategies, and clinical trials are now under evaluation to enhance this therapy’s success and make it available to many more patients.
Acknowledgments
Funding: None.
Footnote
Provenance and Peer Review: This article was commissioned by the Guest Editor (Bruce D. Cheson) for the series “Inaugural Issue” published in Annals of Lymphoma. The article has undergone external peer review.
Conflicts of Interest: Both authors have completed the ICMJE uniform disclosure form (available at http://dx.doi.org/10.21037/aol.2017.10.02). The series “Inaugural Issue” was commissioned by the editorial office without any funding or sponsorship. The authors have no other conflicts of interest to declare.
Ethical Statement: The authors are accountable for all aspects of the work in ensuring that questions related to the accuracy or integrity of any part of the work are appropriately investigated and resolved.
Open Access Statement: This is an Open Access article distributed in accordance with the Creative Commons Attribution-NonCommercial-NoDerivs 4.0 International License (CC BY-NC-ND 4.0), which permits the non-commercial replication and distribution of the article with the strict proviso that no changes or edits are made and the original work is properly cited (including links to both the formal publication through the relevant DOI and the license). See: https://creativecommons.org/licenses/by-nc-nd/4.0/.
References
- Thomas ED, Lochte HL Jr, Lu WC, et al. Intravenous infusion of bone marrow in patients receiving radiation and chemotherapy. N Engl J Med 1957;257:491-6. [Crossref] [PubMed]
- Weiden PL, Flournoy N, Thomas ED, et al. Antileukemic effect of graft-versus-host disease in human recipients of allogeneic-marrow grafts. N Engl J Med 1979;300:1068-73. [Crossref] [PubMed]
- Kolb HJ. Graft-versus-leukemia effects of transplantation and donor lymphocytes. Blood 2008;112:4371-83. [Crossref] [PubMed]
- Barrett DM, Singh N, Liu X, et al. Relation of clinical culture method to T-cell memory status and efficacy in xenograft models of adoptive immunotherapy. Cytotherapy 2014;16:619-30. [Crossref] [PubMed]
- Moss PA, Rosenberg WM, Bell JI. The human T cell receptor in health and disease. Annu Rev Immunol 1992;10:71-96. [Crossref] [PubMed]
- Park TS, Rosenberg SA, Morgan RA. Treating cancer with genetically engineered T cells. Trends Biotechnol 2011;29:550-7. [Crossref] [PubMed]
- Grupp SA, June CH. Adoptive cellular therapy. Curr Top Microbiol Immunol 2011;344:149-72. [Crossref] [PubMed]
- Lee DW, Kochenderfer JN, Stetler-Stevenson M, et al. T cells expressing CD19 chimeric antigen receptors for acute lymphoblastic leukaemia in children and young adults: a phase 1 dose-escalation trial. Lancet 2015;385:517-28. [Crossref] [PubMed]
- Maude SL, Frey N, Shaw PA, et al. Chimeric antigen receptor T cells for sustained remissions in leukemia. N Engl J Med 2014;371:1507-17. [Crossref] [PubMed]
- Davila ML, Brentjens R, Wang X, et al. How do CARs work?: Early insights from recent clinical studies targeting CD19. Oncoimmunology 2012;1:1577-83. [Crossref] [PubMed]
- Davila ML, Sadelain M. Biology and clinical application of CAR T cells for B cell malignancies. Int J Hematol 2016;104:6-17. [Crossref] [PubMed]
- Karlsson H, Svensson E, Gigg C, et al. Evaluation of Intracellular Signaling Downstream Chimeric Antigen Receptors. PLoS One 2015;10:e0144787 [Crossref] [PubMed]
- Davila ML, Papapetrou EP. CARs Move To the Fast Lane. Mol Ther 2014;22:477-8. [Crossref] [PubMed]
- Hudecek M, Sommermeyer D, Kosasih PL, et al. The nonsignaling extracellular spacer domain of chimeric antigen receptors is decisive for in vivo antitumor activity. Cancer Immunol Res 2015;3:125-35. [Crossref] [PubMed]
- Hudecek M, Lupo-Stanghellini MT, Kosasih PL, et al. Receptor affinity and extracellular domain modifications affect tumor recognition by ROR1-specific chimeric antigen receptor T cells. Clin Cancer Res 2013;19:3153-64. [Crossref] [PubMed]
- Rodgers DT, Mazagova M, Hampton EN, et al. Switch-mediated activation and retargeting of CAR-T cells for B-cell malignancies. Proc Natl Acad Sci U S A 2016;113:E459-68. [Crossref] [PubMed]
- Long AH, Haso WM, Orentas RJ. Lessons learned from a highly-active CD22-specific chimeric antigen receptor. Oncoimmunology 2013;2:e23621 [Crossref] [PubMed]
- Kahlon KS, Brown C, Cooper LJ, et al. Specific recognition and killing of glioblastoma multiforme by interleukin 13-zetakine redirected cytolytic T cells. Cancer Res 2004;64:9160-6. [Crossref] [PubMed]
- Zhao Z, Condomines M, van der Stegen SJ, et al. Structural Design of Engineered Costimulation Determines Tumor Rejection Kinetics and Persistence of CAR T Cells. Cancer Cell 2015;28:415-28. [Crossref] [PubMed]
- Kowolik CM, Topp MS, Gonzalez S, et al. CD28 costimulation provided through a CD19-specific chimeric antigen receptor enhances in vivo persistence and antitumor efficacy of adoptively transferred T cells. Cancer Res 2006;66:10995-1004. [Crossref] [PubMed]
- Frigault MJ, Lee J, Basil MC, et al. Identification of chimeric antigen receptors that mediate constitutive or inducible proliferation of T cells. Cancer Immunol Res 2015;3:356-67. [Crossref] [PubMed]
- Guedan S, Chen X, Madar A, et al. ICOS-based chimeric antigen receptors program bipolar TH17/TH1 cells. Blood 2014;124:1070-80. [Crossref] [PubMed]
- Song DG, Powell DJ. Pro-survival signaling via CD27 costimulation drives effective CAR T-cell therapy. Oncoimmunology 2012;1:547-9. [Crossref] [PubMed]
- Song DG, Ye Q, Poussin M, et al. CD27 costimulation augments the survival and antitumor activity of redirected human T cells in vivo. Blood 2012;119:696-706. [Crossref] [PubMed]
- Acuto O, Michel F. CD28-mediated co-stimulation: a quantitative support for TCR signalling. Nat Rev Immunol 2003;3:939-51. [Crossref] [PubMed]
- Ville S, Poirier N, Blancho G, et al. Co-Stimulatory Blockade of the CD28/CD80-86/CTLA-4 Balance in Transplantation: Impact on Memory T Cells? Front Immunol 2015;6:411. [Crossref] [PubMed]
- DeBenedette MA, Wen T, Bachmann MF, et al. Analysis of 4-1BB ligand (4-1BBL)-deficient mice and of mice lacking both 4-1BBL and CD28 reveals a role for 4-1BBL in skin allograft rejection and in the cytotoxic T cell response to influenza virus. J Immunol 1999;163:4833-41. [PubMed]
- Boise LH, Minn AJ, Noel PJ, et al. CD28 costimulation can promote T cell survival by enhancing the expression of Bcl-XL. Immunity 1995;3:87-98. [Crossref] [PubMed]
- Schwarz H, Blanco FJ, von Kempis J, et al. ILA, a member of the human nerve growth factor/tumor necrosis factor receptor family, regulates T-lymphocyte proliferation and survival. Blood 1996;87:2839-45. [PubMed]
- Jung G, Ledbetter JA, Muller-Eberhard HJ. Induction of cytotoxicity in resting human T lymphocytes bound to tumor cells by antibody heteroconjugates. Proc Natl Acad Sci U S A 1987;84:4611-5. [Crossref] [PubMed]
- Azuma M, Cayabyab M, Buck D, et al. CD28 interaction with B7 costimulates primary allogeneic proliferative responses and cytotoxicity mediated by small, resting T lymphocytes. J Exp Med 1992;175:353-60. [Crossref] [PubMed]
- Shuford WW, Klussman K, Tritchler DD, et al. 4-1BB costimulatory signals preferentially induce CD8+ T cell proliferation and lead to the amplification in vivo of cytotoxic T cell responses. J Exp Med 1997;186:47-55. [Crossref] [PubMed]
- Zhou Z, Pollok KE, Kim KK, et al. Functional analysis of T-cell antigen 4-1BB in activated intestinal intra-epithelial T lymphocytes. Immunol Lett 1994;41:177-84. [Crossref] [PubMed]
- van der Windt GJ, Pearce EL. Metabolic switching and fuel choice during T-cell differentiation and memory development. Immunol Rev 2012;249:27-42. [Crossref] [PubMed]
- Yoon J, Schmidt A, Zhang AH, et al. FVIII-specific human chimeric antigen receptor T-regulatory cells suppress T- and B-cell responses to FVIII. Blood 2017;129:238-45. [Crossref] [PubMed]
- Takahashi C, Mittler RS, Vella AT. Cutting edge: 4-1BB is a bona fide CD8 T cell survival signal. J Immunol 1999;162:5037-40. [PubMed]
- Watanabe K, Terakura S, Martens AC, et al. Target antigen density governs the efficacy of anti-CD20-CD28-CD3 zeta chimeric antigen receptor-modified effector CD8+ T cells. J Immunol 2015;194:911-20. [Crossref] [PubMed]
- Frimpong K, Spector SA. Cotransduction of nondividing cells using lentiviral vectors. Gene Ther 2000;7:1562-9. [Crossref] [PubMed]
- Schneider D, Xiong Y, Wu D, et al. A tandem CD19/CD20 CAR lentiviral vector drives on-target and off-target antigen modulation in leukemia cell lines. J Immunother Cancer 2017;5:42. [Crossref] [PubMed]
- Hegde M, Corder A, Chow KK, et al. Combinational targeting offsets antigen escape and enhances effector functions of adoptively transferred T cells in glioblastoma. Mol Ther 2013;21:2087-101. [Crossref] [PubMed]
- Kuramitsu S, Ohno M, Ohka F, et al. Lenalidomide enhances the function of chimeric antigen receptor T cells against the epidermal growth factor receptor variant III by enhancing immune synapses. Cancer Gene Ther 2015;22:487-95. [Crossref] [PubMed]
- Otáhal P, Průková D, Král V, et al. Lenalidomide enhances antitumor functions of chimeric antigen receptor modified T cells. Oncoimmunology 2015;5:e1115940 [Crossref] [PubMed]
- Koneru M, O'Cearbhaill R, Pendharkar S, et al. A phase I clinical trial of adoptive T cell therapy using IL-12 secreting MUC-16(ecto) directed chimeric antigen receptors for recurrent ovarian cancer. J Transl Med 2015;13:102. [Crossref] [PubMed]
- Hurton LV, Singh H, Najjar AM, et al. Tethered IL-15 augments antitumor activity and promotes a stem-cell memory subset in tumor-specific T cells. Proc Natl Acad Sci U S A 2016;113:E7788-97. [Crossref] [PubMed]
- Ruella M, Kenderian SS, Shestova O, et al. The Addition of the BTK Inhibitor Ibrutinib to Anti-CD19 Chimeric Antigen Receptor T Cells (CART19) Improves Responses against Mantle Cell Lymphoma. Clin Cancer Res 2016;22:2684-96. [Crossref] [PubMed]
- Yang J, Riella LV, Chock S, et al. The novel costimulatory programmed death ligand 1/B7.1 pathway is functional in inhibiting alloimmune responses in vivo. J Immunol 2011;187:1113-9. [Crossref] [PubMed]
- Amarnath S, Mangus CW, Wang JC, et al. The PDL1-PD1 axis converts human TH1 cells into regulatory T cells. Sci Transl Med 2011;3:111ra120 [Crossref] [PubMed]
- Cheson BD, Fisher RI, Barrington SF, et al. Recommendations for initial evaluation, staging, and response assessment of Hodgkin and non-Hodgkin lymphoma: the Lugano classification. J Clin Oncol 2014;32:3059-68. [Crossref] [PubMed]
- Wilson WH. Treatment strategies for aggressive lymphomas: what works? Hematology Am Soc Hematol Educ Program 2013;2013:584-90.
- Crump M. Management of Relapsed Diffuse Large B-cell Lymphoma. Hematol Oncol Clin North Am 2016;30:1195-213. [Crossref] [PubMed]
- Davison K, Chen BE, Kukreti V, et al. Treatment outcomes for older patients with relapsed/refractory aggressive lymphoma receiving salvage chemotherapy and autologous stem cell transplantation are similar to younger patients: a subgroup analysis from the phase III CCTG LY.12 trial. Ann Oncol 2017;28:622-7. [PubMed]
- Gisselbrecht C. Futility of relapsed diffuse large B cell lymphoma transplantation? Biol Blood Marrow Transplant 2014;20:1667-9. [Crossref] [PubMed]
- Lim WA, June CH. The Principles of Engineering Immune Cells to Treat Cancer. Cell 2017;168:724-40. [Crossref] [PubMed]
- Kochenderfer JN, Wilson WH, Janik JE, et al. Eradication of B-lineage cells and regression of lymphoma in a patient treated with autologous T cells genetically engineered to recognize CD19. Blood 2010;116:4099-102. [Crossref] [PubMed]
- Turtle CJ, Hanafi LA, Berger C, et al. CD19 CAR-T cells of defined CD4+:CD8+ composition in adult B cell ALL patients. J Clin Invest 2016;126:2123-38. [Crossref] [PubMed]
- Brentjens RJ, Davila ML, Riviere I, et al. CD19-targeted T cells rapidly induce molecular remissions in adults with chemotherapy-refractory acute lymphoblastic leukemia. Sci Transl Med 2013;5:177ra38 [Crossref] [PubMed]
- Sadelain M, Brentjens R, Riviere I, et al. CD19 CAR Therapy for Acute Lymphoblastic Leukemia. Am Soc Clin Oncol Educ Book 2015;e360-3. [Crossref] [PubMed]
- Gattinoni L, Finkelstein SE, Klebanoff CA, et al. Removal of homeostatic cytokine sinks by lymphodepletion enhances the efficacy of adoptively transferred tumor-specific CD8+ T cells. J Exp Med 2005;202:907-12. [Crossref] [PubMed]
- Kochenderfer JN, Dudley ME, Feldman SA, et al. B-cell depletion and remissions of malignancy along with cytokine-associated toxicity in a clinical trial of anti-CD19 chimeric-antigen-receptor-transduced T cells. Blood 2012;119:2709-20. [Crossref] [PubMed]
- Kochenderfer JN, Dudley ME, Kassim SH, et al. Chemotherapy-refractory diffuse large B-cell lymphoma and indolent B-cell malignancies can be effectively treated with autologous T cells expressing an anti-CD19 chimeric antigen receptor. J Clin Oncol 2015;33:540-9. [Crossref] [PubMed]
- Kochenderfer JN, Somerville RPT, Lu T, et al. Lymphoma Remissions Caused by Anti-CD19 Chimeric Antigen Receptor T Cells Are Associated With High Serum Interleukin-15 Levels. J Clin Oncol 2017;35:1803-13. [Crossref] [PubMed]
- Locke FL, Neelapu SS, Bartlett NL, et al. Phase 1 Results of ZUMA-1: A Multicenter Study of KTE-C19 Anti-CD19 CAR T Cell Therapy in Refractory Aggressive Lymphoma. Mol Ther 2017;25:285-95. [Crossref] [PubMed]
- Wang X, Popplewell LL, Wagner JR, et al. Phase 1 studies of central memory-derived CD19 CAR T-cell therapy following autologous HSCT in patients with B-cell NHL. Blood 2016;127:2980-90. [Crossref] [PubMed]
- Turtle CJ, Hanafi LA, Berger C, et al. Immunotherapy of non-Hodgkin's lymphoma with a defined ratio of CD8+ and CD4+ CD19-specific chimeric antigen receptor-modified T cells. Sci Transl Med 2016;8:355ra116 [Crossref] [PubMed]
- Schuster SJ, Svoboda J, Nasta SD, et al. Sustained Remissions Following Chimeric Antigen Receptor Modified T Cells Directed Against CD19 (CTL019) in Patients with Relapsed or Refractory CD19+ Lymphomas. Blood 2015;126:183.
- Abramson JS, Palomba L, Gordon LI, et al. Transcend NHL 001: Immunotherapy with the CD19-Directed CAR T-Cell Product JCAR017 Results in High Complete Response Rates in Relapsed or Refractory B-Cell Non-Hodgkin Lymphoma. Blood 2016;128:4192.
- Kochenderfer JN, Dudley ME, Carpenter RO, et al. Donor-derived CD19-targeted T cells cause regression of malignancy persisting after allogeneic hematopoietic stem cell transplantation. Blood 2013;122:4129-39. [Crossref] [PubMed]
- Porter DL, Antin JH. The graft-versus-leukemia effects of allogeneic cell therapy. Annu Rev Med 1999;50:369-86. [Crossref] [PubMed]
- Brudno JN, Somerville RP, Shi V, et al. Allogeneic T Cells That Express an Anti-CD19 Chimeric Antigen Receptor Induce Remissions of B-Cell Malignancies That Progress After Allogeneic Hematopoietic Stem-Cell Transplantation Without Causing Graft-Versus-Host Disease. J Clin Oncol 2016;34:1112-21. [Crossref] [PubMed]
- Jacoby E, Yang Y, Qin H, et al. Murine allogeneic CD19 CAR T cells harbor potent antileukemic activity but have the potential to mediate lethal GVHD. Blood 2016;127:1361-70. [Crossref] [PubMed]
- MacLeod DT, Antony J, Martin AJ, et al. Integration of a CD19 CAR into the TCR Alpha Chain Locus Streamlines Production of Allogeneic Gene-Edited CAR T Cells. Mol Ther 2017;25:949-61.
- Jensen MC, Popplewell L, Cooper LJ, et al. Antitransgene rejection responses contribute to attenuated persistence of adoptively transferred CD20/CD19-specific chimeric antigen receptor redirected T cells in humans. Biol Blood Marrow Transplant 2010;16:1245-56. [Crossref] [PubMed]
- Till BG, Jensen MC, Wang J, et al. CD20-specific adoptive immunotherapy for lymphoma using a chimeric antigen receptor with both CD28 and 4-1BB domains: pilot clinical trial results. Blood 2012;119:3940-50. [Crossref] [PubMed]
- Ramos CA, Savoldo B, Torrano V, et al. Clinical responses with T lymphocytes targeting malignancy-associated kappa light chains. J Clin Invest 2016;126:2588-96. [Crossref] [PubMed]
- Ramos CA, Ballard B, Zhang H, et al. Clinical and immunological responses after CD30-specific chimeric antigen receptor-redirected lymphocytes. J Clin Invest 2017;127:3462-71. [Crossref] [PubMed]
- Brudno JN, Kochenderfer JN. Toxicities of chimeric antigen receptor T cells: recognition and management. Blood 2016;127:3321-30. [Crossref] [PubMed]
- Lee DW, Gardner R, Porter DL, et al. Current concepts in the diagnosis and management of cytokine release syndrome. Blood 2014;124:188-95. [Crossref] [PubMed]
- Uckun FM, Jaszcz W, Ambrus JL, et al. Detailed studies on expression and function of CD19 surface determinant by using B43 monoclonal antibody and the clinical potential of anti-CD19 immunotoxins. Blood 1988;71:13-29. [PubMed]
- Neelapu SS, Locke FL, Bartlett NL, et al. Axicabtagene ciloleucel (AXI‐CEL; KTE‐C19) in patients with refractory aggresive non-Hodgkin lymphoma (NHL): primamry results of the pivotal trail ZUMA-1. Hematol Oncol 2017;35:28. [Crossref]
- Schuster SJ, Bishop MR, Tam C, et al. Global pivotal phase 2 trial of the CD19-targeted therapy CTL019 in adult patients with relapsed or refractory (R/R) diffuse large B-cell lymphoma (DLBCL)—an interim analysis. Hematol Oncol 2017;35:27. [Crossref]
- Abramson J, Palomba ML, Gordon L, et al. High CR rates in relapsed/refractory (R/R) aggressive B-NHL treated with the CD19-directed CAR T cell product JCAR017 (transcend NHL 001). Hematol Oncol 2017;35:138. [Crossref] [PubMed]
- Jacoby E, Nguyen SM, Fountaine TJ, et al. CD19 CAR immune pressure induces B-precursor acute lymphoblastic leukaemia lineage switch exposing inherent leukaemic plasticity. Nat Commun 2016;7:12320. [Crossref] [PubMed]
- Burnet M. Cancer; a biological approach. I. The processes of control. Br Med J 1957;1:779-86. [Crossref] [PubMed]
- Dunn GP, Bruce AT, Ikeda H, et al. Cancer immunoediting: from immunosurveillance to tumor escape. Nat Immunol 2002;3:991-8. [Crossref] [PubMed]
- Zitvogel L, Tesniere A, Kroemer G. Cancer despite immunosurveillance: immunoselection and immunosubversion. Nat Rev Immunol 2006;6:715-27. [Crossref] [PubMed]
- Mohme M, Riethdorf S, Pantel K. Circulating and disseminated tumour cells - mechanisms of immune surveillance and escape. Nat Rev Clin Oncol 2017;14:155-67. [Crossref] [PubMed]
- Norde WJ, Maas F, Hobo W, et al. PD-1/PD-L1 interactions contribute to functional T-cell impairment in patients who relapse with cancer after allogeneic stem cell transplantation. Cancer Res 2011;71:5111-22. [Crossref] [PubMed]
- Rupp LJ, Schumann K, Roybal KT, et al. CRISPR/Cas9-mediated PD-1 disruption enhances anti-tumor efficacy of human chimeric antigen receptor T cells. Sci Rep 2017;7:737. [Crossref] [PubMed]
- Gargett T, Yu W, Dotti G, et al. GD2-specific CAR T Cells Undergo Potent Activation and Deletion Following Antigen Encounter but can be Protected From Activation-induced Cell Death by PD-1 Blockade. Mol Ther 2016;24:1135-49. [Crossref] [PubMed]
- Evans AG, Rothberg PG, Burack WR, et al. Evolution to plasmablastic lymphoma evades CD19-directed chimeric antigen receptor T cells. Br J Haematol 2015; [Epub ahead of print]. [Crossref] [PubMed]
- Gardner R, Wu D, Cherian S, et al. Acquisition of a CD19-negative myeloid phenotype allows immune escape of MLL-rearranged B-ALL from CD19 CAR-T-cell therapy. Blood 2016;127:2406-10. [Crossref] [PubMed]
- O'Rourke DM, Nasrallah MP, Desai A, et al. A single dose of peripherally infused EGFRvIII-directed CAR T cells mediates antigen loss and induces adaptive resistance in patients with recurrent glioblastoma. Sci Transl Med 2017;9:eaaa0984 [Crossref] [PubMed]
- Anurathapan U, Chan RC, Hindi HF, et al. Kinetics of tumor destruction by chimeric antigen receptor-modified T cells. Mol Ther 2014;22:623-33. [Crossref] [PubMed]
- Sotillo E, Barrett DM, Black KL, et al. Convergence of Acquired Mutations and Alternative Splicing of CD19 Enables Resistance to CART-19 Immunotherapy. Cancer Discov 2015;5:1282-95. [Crossref] [PubMed]
- Scott DW, Gascoyne RD. The tumour microenvironment in B cell lymphomas. Nat Rev Cancer 2014;14:517-34. [Crossref] [PubMed]
- Arita A, McFarland DC, Myklebust JH, et al. Signaling pathways in lymphoma: pathogenesis and therapeutic targets. Future Oncol 2013;9:1549-71. [Crossref] [PubMed]
- Onea AS, Jazirehi AR. CD19 chimeric antigen receptor (CD19 CAR)-redirected adoptive T-cell immunotherapy for the treatment of relapsed or refractory B-cell Non-Hodgkin's Lymphomas. Am J Cancer Res 2016;6:403-24. [PubMed]
Cite this article as: Purev E, Fry TJ. Chimeric antigen receptor T cells for the treatment of lymphoma. Ann Lymphoma 2017;1:7.