Molecular insights into the pathogenesis of follicular lymphoma
Introduction
Follicular lymphomas (FLs) are B-cell neoplasms characterized by clonal proliferation of neoplastic follicle center cells. By far, the most common form is conventional or nodal FL (subsequently referred to as FL in this review). However, in recent years rarer variants have been described that differ in their pathogenesis and clinical features. These new data have led to a rediscovery at the molecular level of the long appreciated morphologic and clinical heterogeneity in FL. This has resulted in the inclusion of newly recognized variants in the 2016 WHO classification, not all of which are associated with the BCL2 translocation, long considered the hallmark of FL (Table 1) (5).
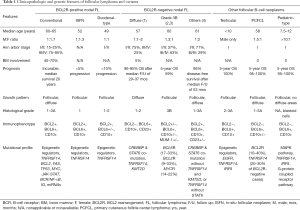
Full table
FL is composed of a mixture of centrocytes and centroblasts, which typically exhibits a follicular growth pattern. FL is subclassified according to the cytological grade based on the proportion of centroblasts. While FL designated as grade 1–2, or grade 3A are positive for the BCL2 rearrangement (BCL2R) in more than 85% of cases, FL grade 3B, is most often negative for BCL2R. A growing body of evidence has suggested that FL grade 3B differs from other forms of FL both biologically and clinically, and is more closely related to diffuse large B-cell lymphoma (5). Therefore, most cases of FL grade 3B emerge as an entity separate from FL grade 1–3A. Optimal recognition of these cases requires integration of histological and genomic features. Other variants of FL that are almost uniformly negative for BCL2R include pediatric-type FL (PTFL), primary cutaneous follicle center lymphoma (PCFCL), and testicular FL (TFL).
In this review we focus on how specific genetic aberrations are related to the biological features of FL, and how they can be incorporated in a clinical setting to aid in differential diagnosis and accurate subclassification. An in-depth discussion of the deregulated molecular pathways and the role of the tumor microenvironment lies beyond the scope of this article and is reviewed elsewhere in this issue.
Conventional nodal FL and t(14;18)-positive variants
In most instances, FL is characterized by an indolent clinical course progressing slowly over years, to eventually become refractory to therapy, or in some case transform into an aggressive lymphoma. Modern therapeutic strategies have considerably improved patient prognosis and the median overall survival is now approaching 20 years; however, advanced stage FL remains virtually incurable (6). A better characterization of the pathogenic mechanisms of FL is important for developing innovative therapies and achieving the ultimate goals of prevention and eradication.
It has become apparent that the pathogenesis of FL is more complex than simply apoptotic resistance caused by BCL2R, implicating genetic and epigenetic alterations, activation of survival pathways, immune evasion and tumor-microenvironment interaction. In the past decade, cytogenetic and high-throughput sequencing technologies have been extensively applied to the study of FL and have provided unprecedented insights into the pathogenesis. Molecular analyses of the tumor in various stages of progression have helped to reveal how the mutational profile evolves during the course of the disease. The findings provide robust evidence for the long-standing hypothesis that FL pathogenesis is a multi-stage and multi-hit process, developing from premalignant lesions and escalating along accumulation of genetic and epigenetic alterations. Secondary genetic events impact the patterns of histological progression, which are diverse. These include diffuse large B-cell lymphoma (7), high grade B-cell lymphoma with double-hit translocations involving MYC and BCL2 (8), B-lymphoblastic leukemia/lymphoma (9), classic Hodgkin lymphoma (10), and histiocytic/dendritic cell sarcoma (11).
Cell of origin
The classical model of FL lymphomagenesis is a multistage and progressive process, whereby t(14;18)(q32;q21) represents the founder event, and clinically significant disease results from a successive accumulation of genetic and epigenetic alterations. It has long been hypothesized that FL derives from transformed germinal center (GC) B cells. This is supported by the findings that the neoplastic cells are often organized in follicles, share cytological, immunophenotypic and gene expression features of GC B cells, as well as display key GC-associated genetic features such as ongoing somatic hypermutation and class switch recombination (12-14). Although the neoplastic cells are able to achieve a state of differentiation closely resembling GC B cells, the acquisition of the genetic hallmark t(14;18) is believed to occur in pre-B cells during a failed variable (V), diversity (D), and joining (J) gene segment rearrangement, which occurs in the marrow (15).
T(14;18)(q32;q21), or on rare occasions its variant t(2;18) (p12;q21) or t(18;22)(q21;q11), can be observed in 80–90% of FL cases (16). These translocations juxtapose the BCL2 oncogene to the IGH, IGK or IGL loci, and place BCL2 under the transcriptional control of immunoglobulin enhancers. This leads to a constitutive expression of the BCL-2 protein from the early stages of B-cell differentiation. Nonmalignant t(14;18)-positive B cells can be detected at low frequency in a large proportion (70%) of healthy individuals over the age of 50, referred to as “FL-like B cells”. However, only a small subset (0.03%) of them will eventually develop FL, years or even decades later (17). The low penetrance and long latency indicate that the BCL2R alone is insufficient for complete transformation, and that additional oncogenic events are required. In fact, t(14;18)-positive memory B cells have the exceptional ability to iteratively reenter GC and engage in multiple rounds of GC reactions upon immunological challenges (18). This is at least partially attributable to the combination of preferential GC reentry of IgM-expressing cells, apoptotic resistance, and uncoupling of differentiation and selection (18). Many such repeated rounds of somatic hypermutation and class-switch recombination confer a high propensity for activation-induced deaminase (AID)-mediated off-target mutagenesis of non-immunoglobulin loci, particularly proto-oncogenes (19,20). Populations with a specific set of genetic events may acquire selective advantage and undergo clonal expansion. The chance to stochastically acquire the particular set of propitious transforming hits must be extremely low. It might take years, or they may never occur in the lifetime of the cells, explaining the wide gap between the incidence of t(14;18)-positive individuals and that of FL.
This model is admittedly oversimplified, and many questions remain unanswered. One important unresolved issue is the drivers, both antigenic and nonantigenic, of GC reentry of (pre)-FL clones. More specifically, whether the process of GC re-entry is driven by chronic exposure to a certain antigen or any antigens in general, and whether FL cells are able to substitute an alternative driver for antigens remain to be clarified (21). Furthermore, although the belief in the importance of BCL-2 in initiation of FL is firmly embedded, the low to modest complete response rate with BCL-2 inhibitors sparks doubt over whether BCL-2 overexpression is required for the maintenance of the tumor (22). It is possible that some fully transformed clones have acquired other anti-apoptotic mechanisms and are no longer dependent on BCL-2. It is therefore important to have predictive biomarkers that can identify patients likely to benefit from anti-BCL-2 therapy. Moreover, mutations specifically required for the emergence of committed FL cancer precursor cells are not yet completely known, and several candidate early hits have been proposed, as will be described in the next section. Lastly, oncogenic hits are not restricted to intrinsic genetic alterations; the interplay between tumor cells and extrinsic factors, such as tumor microenvironment and immune escape, also plays an important role in FL pathogenesis.
Precursors of FL
The possibility of a precursor state to FL was first proposed in the 1990s (23,24). The discovery of non-neoplastic B cells carrying t(14;18) in healthy individuals, later referred to as FL-like B cells, opened a new chapter in the research of FL, suggesting that an uncommitted precursor state may be present with a high prevalence in the general population. Despite an indolent clinical course, FL is typically diagnosed at an advanced stage with generalized lymphadenopathy and frequent bone marrow involvement. This seemingly paradoxical observation suggests a protracted, insidious preclinical phase. Therefore, prevention and treatment of FL might benefit from identification and therapeutic targeting of its early precursors. In fact, several lesions have now been recognized as putative early stages of FL lymphomagenesis, including in-situ follicular neoplasia (ISFN), partial involvement by FL, and duodenal-type FL (5).
ISFN, previously designated as FL in situ, is defined as colonization of GCs by clonal B cells carrying the BCL2R in an otherwise reactive-appearing lymph node (25). It has been reported in 2–3% of unselected reactive lymph nodes (26), and the risk of subsequent FL is very low (<5%) (25). It is usually discovered as an incidental finding in the setting of reactive follicular hyperplasia or other forms of usually B-cell lymphoma. ISFN is usually not suspected on H&E staining and is mainly detected by immunohistochemistry, which shows centrocytes strongly expressing BCL-2 and GC markers, in the absence of disruption of follicular architecture and interfollicular infiltration (25). ISFN is distinguished from partial involvement by FL, as the latter is a BCL2-positive follicular proliferation that alters the architecture and is readily evident on routine H&E stains. By array comparative genomic hybridization (CGH), ISFN has a very low level of genomic aberrations beyond the BCL2 translocation. Interestingly, cases of partial involvement also show a relatively low level of genomic aberrations (27).
Duodenal-type FL is often discovered incidentally, and typically manifests as multiple small polyps in the second portion of the duodenum. The disease has an indolent clinical course and is associated with excellent survival. The follicles consist almost entirely of centrocytes, with an immunophenotype similar to that of nodal FL, and a low proliferation index (28). Infiltration of the lamina propria beyond the follicles is common, but the disease tends to remain localized to the intestinal mucosa with low risk (<10%) of progression to nodal disease.
ISFN and duodenal-type FL resemble nodal FL in their follicular growth pattern, cytological features and immunophenotype. However, they have a favorable prognosis, and low rates of progression to overt FL. Nevertheless, sensitive PCR-based testing may indicate that patients with ISFN have FL-like B cells circulating in the peripheral blood (29). A high content of circulating FL-like B cells indicates an increased risk for eventual FL (30).
The molecular basis of pathogenesis of FL
In recent years, studies have characterized the nature and temporal ordering of mutations in FL at different developmental time points, to identify additional “hits” that cooperate with t(14;18) in tumor initiation and progression. The molecular understanding of FL and its early lesions has had a profound impact on our understanding of its pathogenesis.
Mutations in epigenetic regulators and chromatin remodelers, such as CREBBP, MLL2, EP300, EZH2, and MEF2B, have emerged as a hallmark of FL. These mutations are present in approximately 85% of cases, and mutations of multiple different epigenetic regulators frequently occur in the same tumor (31). The findings suggest that they likely occur early in FL development, and epigenetic alteration is an important pathogenic mechanism for FL (31,32). Our understanding of the pathogenic role of epigenetic dysregulation in FL is still at its early stage. Many chromatin remodelers possess the ability to modify not only histones, but also non-histone proteins. Two examples of targeted non-histone proteins are TP53 and BCL-6; dysregulated acetylation of these two proteins allows constitutive activation of BCL-6 oncoprotein and decreased TP53 tumor suppressor activity, leading to an increased tolerance for DNA damage in the context of diminished apoptotic and cell cycle arrest responses (33,34). Furthermore, some of the targeted non-histone proteins themselves can regulate epigenetic and transcriptional programs. Given the broad and multilayered influence of these epigenetic regulators on transcriptional regulation, it is difficult to predict which cellular pathways are critically affected in lymphomagenesis. One consequence of altered gene expression profile might be freezing the cells at a functional state of GC B cells, known to have a “mutator phenotype” with high proliferation rates and “physiologic” genomic instability, which facilitates the acquisition of additional mutations. The most common additional “hits” include mutations in TNFRSF14, some tumor suppressors, miRNA, as well as genes involved in JAK-STAT, B-cell receptor/NF-κB and apoptosis signaling pathways [reviewed in (35)]. In addition to their roles in modification of chromatin structure, some of the epigenetic regulators, such as CREBBP and EP300, also serve as transcriptional co-activators, play an essential role in DNA damage response, and are considered as tumor suppressors (36). Therefore, mutations of these genes likely have broad phenotypic consequences extending beyond their direct effects on epigenetic and transcriptional programming.
Recent studies have also provided strong support at the molecular level to the existence of precursors or early stages of FL. Overall, the mutational profiles of ISFN and duodenal-type FL are similar to that of clinically significant FL, but less complex (37). In common with FL, the early lesions carry BCL2R and may harbor alterations in TNFRSF14 and epigenetic regulators such as EZH2 and CREBBP, with similar mutation type, pattern and distribution (38). However, they show lower frequencies of large losses, chromosomal copy-number abnormalities, and KMT2D mutations (27,37,39). The presence of secondary genetic abnormalities in ISFN suggests that genomic instability is already at work, although insufficient for full transformation. Therefore, ISFN is best regarded as an uncommitted precursor of FL from both the clinical and biologic perspectives. Duodenal-type FL shares genomic properties with ISFN, but likely remains confined to the intestinal mucosa based on homing receptors, and response to antigen in this specialized immune environment (38,39).
BCL2R negative nodal FL
Although the classical FL pathogenesis model has gained growing support through molecular studies, it provides an incomplete picture of the pathogenic pathways of FL. It has become clear that the genetic evolution of FL does not follow a single linear sequence of certain mutations; rather, it evolves through multiple independent or convergent pathways. Ten to fifteen percent of conventional FL cases do not carry the hallmark BCL2 translocation (16). These cases are most common in females, often presents in early stages at diagnosis, and has an excellent prognosis (4). In contrast to the extensive investigation of the mutational landscape of t(14;18)-positive FL, there have been more limited systematic studies on the genetic events in t(14;18)-negative (nodal) FL. Nonetheless, the currently available data reveal two important findings. First, overall, t(14;18)-negative FL carries similar copy number alterations and gene mutations to those reported in t(14;18)-positive FL, although with different frequencies. Therefore, based on its close relatedness to t(14;18)-positive FL emerging at the molecular level, t(14;18)-negative FL is best regarded as a genetic subgroup of conventional FL, rather than a biologically unrelated disease. Second, t(14;18)-negative FL is heterogenous at the genetic level. The contrast between the genetic homogeneity of t(14;18)-positive FL and heterogeneity of t(14;18)-negative FL suggests that the pattern of secondary genetic changes in FL largely depends on the nature of the primary event. BCL-2 overexpression provides a fertile ground for certain mutations; in the absence of t(14;18), the founder and key secondary mutations are possibly acquired, at a slower pace, through multiple different mechanisms or simply stochastically; hence the lower incidence and higher heterogeneity of this group.
The most prominent cytogenetic changes in t(14;18)-negative FL are numeric and structural alterations of chromosome 3. In particular, alterations involving the 3q27 locus, the site of the BCL6 gene, are observed in approximately 30% of cases (40). This promiscuous locus can be translocated with a wide variety of partners, which lead to its anomalous upregulation, by disrupting its negative autoregulation, blocking its downregulation by CD40 signaling, or introducing a strong enhancer signal (41,42). Alternatively, BCL6 amplification and mutations, as well as 3q27 gains, can also lead to BCL-6 overexpression. BCL-6 is known as a master transcription regulator involved in GC formation, and protecting GC B cells against apoptosis during GC reaction (43,44). It remains unclear whether BCL6 aberrations represent an early founder mutation like BCL2, a secondary driver, or simply a marker of genomic instability but less directly related to the transformation process. The answer to this question is important for designing BCL-6 targeted therapy. In general, FL lacking t(14;18) but carrying BCL6 abnormalities tend to be diagnosed at more advanced clinical stages, present with higher histologic grades and less frequent CD10 expression (45), and are more likely to show a complex genetic profile with higher chance of carrying alterations in 17p (TP53) (4). Some authors believe that FL with BCL6 translocations may be more prone to subsequent early transformation and associated with poorer prognosis (46). However, larger prospective studies are needed to confirm the findings. This subgroup is exemplified by the cases reported by Karube and colleagues in 2007 (47). These cases are most frequently encountered in the elderly, and often exhibit a follicular growth pattern and high-grade morphology (grade 3A and 3B). The atypical follicles have a peculiar immunophenotype, positive for MUM-1 but negative for CD10. BCL6 aberrations (translocation or amplification) are detected in the vast majority (88%) of the cases. Notably, compared to cases with BCL6 translocations, those with BCL6 amplification/3q27 gain showed more frequent grade 3 morphology, and higher BCL-2 and MUM-1 expression, often in combination with BCL2 gene amplification/18q21 gain (90%) (48). These observations may suggest a dose-dependent effect of BCL-6. Alternatively, patients with different types of BCL6 aberrations may belong to distinct molecular entities.
The most frequently mutated gene in t(14;18)-negative FL is STAT6 (57%), which usually co-occurred with TNFRSF14 (39%) and/or CREBBP (49%) alterations. KMT2D is frequently mutated as well (27%) (4). Of note, these aberrations also represent frequent secondary aberrations in t(14;18)-positive FL. Genetic alterations appear to influence clinical, pathological and immunophenotypic features, which, reversely, may predict underlying genetic alterations. For instance, in contrast to BCL6 translocated cases that usually show a follicular growth pattern, cases carrying both STAT6 and TNFRSF14 alterations are associated with a diffuse growth pattern. Furthermore, CD23 expression can often predict the presence of STAT6 mutations. As suggested in a recent study, t(14;18)-negative FL can be roughly clustered into different subgroups based on the presence and coexistence of different mutations (4). Further studies are needed to define more homogeneous subgroups among these patients.
An ideal classification should contain diseases that are clearly defined, clinically distinctive, and mutually exclusive. The ultimate value of classifying disease subgroups is realized when they are connected to clinically meaningful outcomes, which are often strongly related to the underlying mechanisms. The clinicopathological and genetic heterogeneity of t(14;18)-negative FL suggests that a simple system of subclassification may not be sufficient. A classification strategy, which is not only based on morphological features but also is supplemented by clinically significant mutations, may help identify subgroups of FL that share common pathogenic pathways although morphologically appearing distinct and those superficially looking similar but in fact mechanistically different. We will elaborate on this aspect below using the diffuse FL variant as an example.
Diffuse FL variant
This unique variant of low-grade nodal FL was first described in 2009 by Katzenberger et al. (1). This variant is characterized by a predominantly diffuse architecture and mixed centrocytic/centroblastic cytology, with expression of at least one GC marker, CD23 co-expression, and weak to absent BCL-2 staining (Figure 1A,B,C,D,E,F). Many of the cases exhibit unique clinical features, including low clinical stage, localized inguinal lymph node involvement, and favorable prognosis. Molecular characterization revealed a near-uniform presence of CREBBP and STAT6 co-mutation and a high frequency of 1p36/TNFRSF14 abnormalities and KMT2D mutations, in the context of absent t(14;18) (49). Additionally, mutations in BCL2, HEATR6, LAMA5 and VPS13B, as well as an enrichment of mutated genes coding for basement membrane proteins, have also been reported in smaller series (50). Overall, the mutational profile of this variant shows a high degree of similarity to that of conventional FL. Furthermore, the gene expression analysis showed a profile within the spectrum of FL, albeit clustering as a distinct group (1).
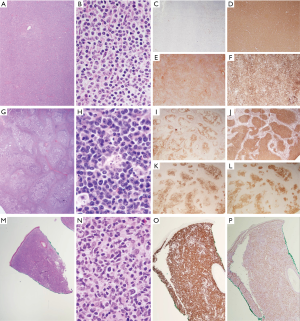
The understanding of the molecular mechanism of this diffuse variant broadens and deepens our understanding of FL pathogenesis, and aids accurate subclassification. Although t(14;18)-positive FL and the diffuse variant differ in the founder aberrations at the starting point of lymphomagenesis, their secondary alterations appear to be largely similar, and likely converge to key oncogenic pathways. In other words, certain mutations can function as (secondary) drivers/accelerators in t(14;18)-positive FL, but founders in the absence of t(14;18). These findings suggest that the diffuse variant represents a morphological variant of conventional FL, rather than a biologically distinct disease, despite a diffuse growth pattern and lack of BCL2R, thus confirming the WHO classification (5). This concept is further supported by the findings that some FL cases carrying co-mutation of STAT6 and CREBBP, in the absence of TNFRSF14 and EZH2, show a follicular growth pattern, but otherwise share many features with the diffuse variant including female predilection, inguinal location, CD23 expression, and excellent prognosis (4). Therefore, instead of segregating cases based solely on growth patterns (diffuse vs. follicular), it may be more appropriate to categorize these cases and the diffuse variant into the same genetic subgroup based on the presence of co-mutation of STAT6 and CREBBP, in the context of similar clinicopathological features.
The genetic and mutational profile can be a useful tool in diagnostically challenging cases. For instance, CD10-negative diffuse FL variant occasionally occurs, making the distinction from nodal marginal zone lymphoma particularly difficult (1). Identification of the 1p36/TNFRSF14 abnormalities along with CREBBP and STAT6 mutations would support a diagnosis of a t(14;18)-negative FL. Additionally, the diffuse FL variant shows gains and amplification of the REL locus (51), an alteration frequently present in GC-derived B-cell lymphoma, and lacks trisomies 3, 7 and 18, as well as NOTCH2 mutations, which are characteristic of marginal zone lymphoma (4,52,53).
Other Follicular B-cell neoplasms, unrelated to conventional FL
Pediatric-type follicular lymphoma (PTFL)
PTFL is a newly incorporated entity in the 2016 WHO classification, exhibiting unique clinical and histological characteristics distinct from FL (5). It primarily affects children and adolescents with a clear male predominance (10:1), in contrast to FL that is exceedingly rare in this age group. The vast majority of patients present with localized stage I cervical lymphadenopathy. Histologically, the atypical follicles are composed of monotonous, medium-sized blastoid cells, often with a “starry-sky” appearance and a moderate to high proliferative rate (Figure 1G,H,I,J,K,L). Despite the high-grade histological appearance, its prognosis is excellent; patients usually achieve a long-term complete remission, without additional therapy beyond surgical excision. The almost invariably benign behavior of PTFL has led to the question whether it truly represents a malignant lymphoma rather than atypical lymphoid hyperplasia.
Recent studies revealed recurrent genomic changes in PTFL, which confirms the neoplastic, rather than reactive, nature of this process. Critically, the results have shown that PTFL differs genetically from FL. Overall, PTFL has a low level of genomic complexity (54,55). Structural chromosomal aberrations are uncommon in PTFL, and BCL2, BCL6, MYC, and IRF4 rearrangements are absent in PTFL (56). These findings are in keeping with a low malignant potential of PTFL, which correlates well with its favorable prognosis. Furthermore, there is only minimal overlap between the mutational profiles of PTFL and FL. Mutations in epigenetic modifiers, a hallmark of FL, are uncommon in PTFL. KMT2D is the most frequently mutated histone-modifying gene in PTFL and is seen in only 16% of cases. Others such as CREBBP, EP300, MEF2B, and EZH2 are seldomly affected (55). However, a very recent study identified novel mutations in two epigenetic regulators NSD1 and RSF1 in a subset of PTFL cases, using whole exome deep sequencing (57). This raises the possibility that mutations in epigenetic modifiers may occur more frequently in PTFL than previously appreciated. Future detailed genome-wide studies on larger cohorts are needed to further explore this possibility. Additionally, STAT6 mutations are usually not present in PTFL. Aberrations in MAP2K1 and 1p36/TNFRSF14 are the most common genetic changes in PTFL, each observed in 30–70% of the cases (54,55,58). A recurrent loss-of-function mutation in IRF8, a tumor suppressor, at the hotspot p.K66R, was recently described in 50% (3 out of 6) of PTFL patients in a small cohort (59), which needs to be confirmed in a larger cohort. Interestingly, TNFRSF14 mutations often coexist with IRF8 mutations, while mutations in these two genes do not occur together with MAP2K1 mutations in the majority of the cases. These findings may suggest two divergent pathways in PTFL lymphomagenesis: one through activation of the MAPK pathway, and the other involving TNFRSF14 and IRF8. The involvement of MAPK pathway was further confirmed by a recent study, which identified few novel mutations that have not been described previously, mostly belonging to two highly interlaced signaling pathways: “negative regulation of MAPK” and “G-protein coupled receptor” (57). Taken together, these findings define PTFL as a biologically distinct form of lymphoma, as categorized in the current WHO classification.
Testicular follicular lymphoma
TFL typically affects children, but also rarely occurs in adults (60,61). Similar to PTFL, it is characterized by high histologic grades (usually grade 3A), lack of BCL-2 expression and t(14;18), a moderate to high proliferative rate, localized disease, and a good prognosis with sustained complete remission following surgical excision alone (62,63). The data suggest that, similar to other childhood tumors, FL in pediatric patients, irrespective of the primary site, has a different molecular pathogenesis from the adult counterparts.
In one recent study, whole exome sequencing was performed on two patients and targeted sequencing of TNFRSF14 was performed on one patient with TFL. The results from this small cohort revealed somatic mutations that overlap with those in both FL and PTFL, involving MAPK pathway (EGFR), epigenetic modifiers (EZH2, KMT2D), TNFRSF14, and IRF8 (57). Further studies with larger cohorts are needed to establish the detailed molecular picture of TFL.
Primary cutaneous follicle center lymphoma
PCFCL is a tumor of neoplastic follicle center cells, typically presenting as localized skin lesions in a single region, particularly in the head and neck area or the trunk. Histologically, a nodular, diffuse, or mixed growth pattern is seen, composed of a variable proportion of centrocytes, which are often large, with few centroblasts. The atypical cells express BCL-6, but CD10 and BCL-2 expression is uncommon (Figure 1M,N,O,P). Although PCFCL share some histologic and immunophenotypic features with its node-based counterpart, it carries a much better prognosis with a 5-year survival rate approaching 100% following local treatment (64,65).
PCFCL has a heterogeneous genetic background: BCL2R are seen in 10–40% of cases, while 1p36/TNFRSF14 abnormalities are mutually exclusive with BCL2R and occur in 20–30% of BCL2-negative patients (66-68). The vast majority of the BCL2R-negative cases also demonstrate high immunohistochemical expression of EZH2, albeit in the absence of tested EZH2 hotspot mutations (68). PCFCL appears to harbor only a low level of deleterious genetic variations. Rearrangements involving BCL6, MYC, and MALT1 genes are rare (69). Hypermethylation in CDKN2A and CDKN2B and aberrant somatic hypermutation in BCL6, common abnormalities in primary cutaneous large B-cell lymphoma, leg type, are only seen in a minority of PCFCL cases (70).
Few important questions remain incompletely answered regarding the classification of PCFCL. First, although PCFCL in general has been considered a distinct entity that is unrelated to conventional FL, findings of a recent study seem to cast doubts on this notion (71). This study showed that mutations detected in PCFCL were very similar to those observed in FL, in particular FL negative for BCL2R. The commonly mutated genes include 1p36/TNFRSF14 aberrations (50%), epigenetic regulators [CREBBP (25%), KMT2D (21%), EP300 (18%)], NF-κB pathway [TNFAIP3 (25%)], JAK-STAT pathway [SOCS1 (20%), STAT6 (17%)], and FOXO1 (17%). Therefore, conceptually, it may be more appropriate to consider PCFCL as a clinicopathological variant of BCL2R-negative nodal FL, rather than a genetically unrelated entity like PTFL and TFL. The few cases carrying BCL2R may represent a different biological entity and should be segregated from BCL2R-negative cases. A large cohort comparing the prognosis and mutational landscape between these two groups may be informative.
The diagnosis of PCFCL can be problematic in some occasions, including the absolute distinction between PCFCL and systemic/nodal FL with secondary cutaneous involvement. In current practice, the distinction mainly depends on the assessment of systemic disease. In fact, these two entities are different in the combinatorial pattern of 1p36/TNFRSF14 abnormalities and BCL2R: these two types of aberrations are mutually exclusive in PCFCL, while in nodal FL, 1p36/TNFRSF14 abnormalities usually present as a secondary change to t(14;18). Therefore, testing for both the BCL2R and 1p36/TNFRSF14 abnormalities may serve as a useful diagnostic tool. More distinguishing criteria have been proposed by a recent study, which demonstrated that the presence of BCL2R, mutations in 2 or more chromatin-modifying genes, and a low proliferation index (Ki67 <30%) were associated with a high likelihood of concurrent or future systemic involvement (72). Furthermore, the absence of BCL6 translocations, MYD88 mutations, and inactivation of CDKN2A and CDKN2B favor a diagnosis of PCFCL over primary cutaneous large B-cell lymphoma, leg type. Additionally, the presence of genetic abnormalities may assist in distinction of PCFCL from lymphoid hyperplasia, with the latter typically not displaying genomic aberrations.
Conclusions
The recent advances obtained via genomic profiling efforts are expanding our understanding of the pathogenesis of FL, and have opened up potential new avenues for improving diagnosis and management of FL. Mutations in epigenetic regulators have gained increased attention as one genetic hallmark of FL. Elucidation of the precise oncogenic mechanisms by which mutations in epigenetic regulators contribute to FL pathogenesis remains a complex and unresolved task. Another highly recurrent genetic alteration is 1p36/TNFRSF14 abnormality, which is seen across different subtypes of FL, irrespective of the presence of t(14;18). This highlights the importance of immune escape in the pathogenesis of FL.
The clinicopathologic heterogeneity of neoplasms derived from follicular B cells corresponds to distinct molecular subtypes with different biological properties. Notably, several variants are associated with localized disease and excellent prognosis. The new information further validates the incorporation of genetic aberrations in FL diagnosis and classification. In the vast majority of cases, a confident diagnosis can be made based on morphology and histochemistry alone. When in doubt, FISH testing for BCL2R can be performed. In the absence of BCL2R, next-generation sequencing or copy number variation may follow to look for specific alterations. Genomic studies have led to a recognition of new entities derived from follicular B-lymphocytes. Additionally, there is overlap in the genetic alterations between FL and other B-cell lymphomas, as well as among different FL subtypes. Any molecular results should not be interpreted in isolation but in the context of clinicopathological findings, with careful examination of histological details. Mechanistic heterogeneity is often reflected in sometimes subtle, but noticeable, pathological features. Such differences can be used to identify disease subtypes that are more recognizable as molecular-defined entities.
Acknowledgments
Funding: None.
Footnote
Provenance and Peer Review: This article was commissioned by the Guest Editors (Mark Roschewski, Carla Casulo) for the series “Follicular Lymphoma” published in Annals of Lymphoma. The article has undergone external peer review.
Conflicts of Interest: All authors have completed the ICMJE uniform disclosure form (available at http://dx.doi.org/10.21037/aol-20-49). The series “Follicular Lymphoma” was commissioned by the editorial office without any funding or sponsorship. The authors have no other conflicts of interest to declare.
Ethical Statement: The authors are accountable for all aspects of the work in ensuring that questions related to the accuracy or integrity of any part of the work are appropriately investigated and resolved.
Open Access Statement: This is an Open Access article distributed in accordance with the Creative Commons Attribution-NonCommercial-NoDerivs 4.0 International License (CC BY-NC-ND 4.0), which permits the non-commercial replication and distribution of the article with the strict proviso that no changes or edits are made and the original work is properly cited (including links to both the formal publication through the relevant DOI and the license). See: https://creativecommons.org/licenses/by-nc-nd/4.0/.
References
- Katzenberger T, Kalla J, Leich E, et al. A distinctive subtype of t(14;18)-negative nodal follicular non-Hodgkin lymphoma characterized by a predominantly diffuse growth pattern and deletions in the chromosomal region 1p36. Blood 2009;113:1053-61. [Crossref] [PubMed]
- Harris NL, Kluin P. Follicular lymphoma grade 3B: is it a real disease? Haematologica 2011;96:1244-6. [Crossref] [PubMed]
- Hans CP, Weisenburger DD, Vose JM, et al. A significant diffuse component predicts for inferior survival in grade 3 follicular lymphoma, but cytologic subtypes do not predict survival. Blood 2003;101:2363-7. [Crossref] [PubMed]
- Nann D, Ramis-Zaldivar JE, Muller I, et al. Follicular lymphoma t(14;18)-negative is genetically a heterogeneous disease. Blood Adv 2020;4:5652-65. [Crossref] [PubMed]
- Swerdlow SH CE, Harris NL, Jaffe ES, et al. WHO Classification of Tumours of Haematopoietic and Lymphoid Tissues. Revised Fourth Edition. 4th ed. Lyon, France: International Agency for Research on Cancer; 2017.
- Provencio M, Sabin P, Gomez-Codina J, et al. Impact of treatment in long-term survival patients with follicular lymphoma: A Spanish Lymphoma Oncology Group registry. PLoS One 2017;12:e0177204 [Crossref] [PubMed]
- Davies AJ, Rosenwald A, Wright G, et al. Transformation of follicular lymphoma to diffuse large B-cell lymphoma proceeds by distinct oncogenic mechanisms. Br J Haematol 2007;136:286-93. [Crossref] [PubMed]
- Bischin AM, Dorer R, Aboulafia DM. Transformation of Follicular Lymphoma to a High-Grade B-Cell Lymphoma With MYC and BCL2 Translocations and Overlapping Features of Burkitt Lymphoma and Acute Lymphoblastic Leukemia: A Case Report and Literature Review. Clin Med Insights Blood Disord 2017;10:1179545X17692544.
- Sun X, Gordon LI, Peterson LC. Transformation of follicular lymphoma to acute lymphoblastic leukemia. Arch Pathol Lab Med 2002;126:997-8. [Crossref] [PubMed]
- Menon MP, Hutchinson L, Garver J, et al. Transformation of follicular lymphoma to Epstein-Barr virus-related Hodgkin-like lymphoma. J Clin Oncol 2013;31:e53-6. [Crossref] [PubMed]
- Brunner P, Rufle A, Dirnhofer S, et al. Follicular lymphoma transformation into histiocytic sarcoma: indications for a common neoplastic progenitor. Leukemia 2014;28:1937-40. [Crossref] [PubMed]
- Victora GD, Dominguez-Sola D, Holmes AB, et al. Identification of human germinal center light and dark zone cells and their relationship to human B-cell lymphomas. Blood 2012;120:2240-8. [Crossref] [PubMed]
- Gagyi E, Balogh Z, Bodor C, et al. Somatic hypermutation of IGVH genes and aberrant somatic hypermutation in follicular lymphoma without BCL-2 gene rearrangement and expression. Haematologica 2008;93:1822-8. [Crossref] [PubMed]
- Roulland S, Navarro JM, Grenot P, et al. Follicular lymphoma-like B cells in healthy individuals: a novel intermediate step in early lymphomagenesis. J Exp Med 2006;203:2425-31. [Crossref] [PubMed]
- Cleary ML, Sklar J. Nucleotide sequence of a t(14;18) chromosomal breakpoint in follicular lymphoma and demonstration of a breakpoint-cluster region near a transcriptionally active locus on chromosome 18. Proc Natl Acad Sci U S A 1985;82:7439-43. [Crossref] [PubMed]
- Vaandrager JW, Schuuring E, Raap T, et al. Interphase FISH detection of BCL2 rearrangement in follicular lymphoma using breakpoint-flanking probes. Genes Chromosomes Cancer 2000;27:85-94. [Crossref] [PubMed]
- Schüler F, Dolken L, Hirt C, et al. Prevalence and frequency of circulating t(14;18)-MBR translocation carrying cells in healthy individuals. Int J Cancer 2009;124:958-63. [Crossref] [PubMed]
- Sungalee S, Mamessier E, Morgado E, et al. Germinal center reentries of BCL2-overexpressing B cells drive follicular lymphoma progression. J Clin Invest 2014;124:5337-51. [Crossref] [PubMed]
- Rossi D, Berra E, Cerri M, et al. Aberrant somatic hypermutation in transformation of follicular lymphoma and chronic lymphocytic leukemia to diffuse large B-cell lymphoma. Haematologica 2006;91:1405-9. [PubMed]
- Halldórsdóttir AM, Fruhwirth M, Deutsch A, et al. Quantifying the role of aberrant somatic hypermutation in transformation of follicular lymphoma. Leuk Res 2008;32:1015-21. [Crossref] [PubMed]
- Linley A, Krysov S, Ponzoni M, et al. Lectin binding to surface Ig variable regions provides a universal persistent activating signal for follicular lymphoma cells. Blood 2015;126:1902-10. [Crossref] [PubMed]
- Davids MS, Roberts AW, Seymour JF, et al. Phase I First-in-Human Study of Venetoclax in Patients With Relapsed or Refractory Non-Hodgkin Lymphoma. J Clin Oncol 2017;35:826-33. [Crossref] [PubMed]
- Limpens J, de Jong D, van Krieken JH, et al. Bcl-2/JH rearrangements in benign lymphoid tissues with follicular hyperplasia. Oncogene 1991;6:2271-6. [PubMed]
- Limpens J, Stad R, Vos C, et al. Lymphoma-associated translocation t(14;18) in blood B cells of normal individuals. Blood 1995;85:2528-36. [Crossref] [PubMed]
- Jegalian AG, Eberle FC, Pack SD, et al. Follicular lymphoma in situ: clinical implications and comparisons with partial involvement by follicular lymphoma. Blood 2011;118:2976-84. [Crossref] [PubMed]
- Henopp T, Quintanilla-Martinez L, Fend F, et al. Prevalence of follicular lymphoma in situ in consecutively analysed reactive lymph nodes. Histopathology 2011;59:139-42. [Crossref] [PubMed]
- Mamessier E, Song JY, Eberle FC, et al. Early lesions of follicular lymphoma: a genetic perspective. Haematologica 2014;99:481-8. [Crossref] [PubMed]
- Schmatz AI, Streubel B, Kretschmer-Chott E, et al. Primary follicular lymphoma of the duodenum is a distinct mucosal/submucosal variant of follicular lymphoma: a retrospective study of 63 cases. J Clin Oncol 2011;29:1445-51. [Crossref] [PubMed]
- Cheung MC, Bailey D, Pennell N, et al. In situ localization of follicular lymphoma: evidence for subclinical systemic disease with detection of an identical BCL-2/IGH fusion gene in blood and lymph node. Leukemia 2009;23:1176-9. [Crossref] [PubMed]
- Roulland S, Kelly RS, Morgado E, et al. t(14;18) Translocation: A predictive blood biomarker for follicular lymphoma. J Clin Oncol 2014;32:1347-55. [Crossref] [PubMed]
- Green MR, Kihira S, Liu CL, et al. Mutations in early follicular lymphoma progenitors are associated with suppressed antigen presentation. Proc Natl Acad Sci U S A 2015;112:E1116-25. [Crossref] [PubMed]
- Bödör C, Grossmann V, Popov N, et al. EZH2 mutations are frequent and represent an early event in follicular lymphoma. Blood 2013;122:3165-8. [Crossref] [PubMed]
- Pasqualucci L, Dominguez-Sola D, Chiarenza A, et al. Inactivating mutations of acetyltransferase genes in B-cell lymphoma. Nature 2011;471:189-95. [Crossref] [PubMed]
- Glozak MA, Sengupta N, Zhang X, et al. Acetylation and deacetylation of non-histone proteins. Gene 2005;363:15-23. [Crossref] [PubMed]
- Huet S, Sujobert P, Salles G. From genetics to the clinic: a translational perspective on follicular lymphoma. Nat Rev Cancer 2018;18:224-39. [Crossref] [PubMed]
- Goodman RH, Smolik S. CBP/p300 in cell growth, transformation, and development. Genes Dev 2000;14:1553-77. [PubMed]
- Schmidt J, Salaverria I, Haake A, et al. Increasing genomic and epigenomic complexity in the clonal evolution from in situ to manifest t(14;18)-positive follicular lymphoma. Leukemia 2014;28:1103-12. [Crossref] [PubMed]
- Jaffe ES, Quintanilla-Martinez L. t(14;18)-positive B cells: is it seed or soil? Blood 2018;132:1631-2. [Crossref] [PubMed]
- Hellmuth JC, Louissaint A Jr, Szczepanowski M, et al. Duodenal-type and nodal follicular lymphomas differ by their immune microenvironment rather than their mutation profiles. Blood 2018;132:1695-702. [Crossref] [PubMed]
- Gu K, Fu K, Jain S, et al. t(14;18)-negative follicular lymphomas are associated with a high frequency of BCL6 rearrangement at the alternative breakpoint region. Mod Pathol 2009;22:1251-7. [Crossref] [PubMed]
- Pasqualucci L, Migliazza A, Basso K, et al. Mutations of the BCL6 proto-oncogene disrupt its negative autoregulation in diffuse large B-cell lymphoma. Blood 2003;101:2914-23. [Crossref] [PubMed]
- Saito M, Gao J, Basso K, et al. A signaling pathway mediating downregulation of BCL6 in germinal center B cells is blocked by BCL6 gene alterations in B cell lymphoma. Cancer Cell 2007;12:280-92. [Crossref] [PubMed]
- Fukuda T, Yoshida T, Okada S, et al. Disruption of the Bcl6 gene results in an impaired germinal center formation. J Exp Med 1997;186:439-48. [Crossref] [PubMed]
- Phan RT, Dalla-Favera R. The BCL6 proto-oncogene suppresses p53 expression in germinal-centre B cells. Nature 2004;432:635-9. [Crossref] [PubMed]
- Díaz-Alderete A, Doval A, Camacho F, et al. Frequency of BCL2 and BCL6 translocations in follicular lymphoma: relation with histological and clinical features. Leuk Lymphoma 2008;49:95-101. [Crossref] [PubMed]
- Akasaka T, Lossos IS, Levy R. BCL6 gene translocation in follicular lymphoma: a harbinger of eventual transformation to diffuse aggressive lymphoma. Blood 2003;102:1443-8. [Crossref] [PubMed]
- Karube K, Guo Y, Suzumiya J, et al. CD10-MUM1+ follicular lymphoma lacks BCL2 gene translocation and shows characteristic biologic and clinical features. Blood 2007;109:3076-9. [Crossref] [PubMed]
- Karube K, Ying G, Tagawa H, et al. BCL6 gene amplification/3q27 gain is associated with unique clinicopathological characteristics among follicular lymphoma without BCL2 gene translocation. Mod Pathol 2008;21:973-8. [Crossref] [PubMed]
- Xian RR, Xie Y, Haley LM, et al. CREBBP and STAT6 co-mutation and 16p13 and 1p36 loss define the t(14;18)-negative diffuse variant of follicular lymphoma. Blood Cancer J 2020;10:69. [Crossref] [PubMed]
- Zamò A, Pischimarov J, Horn H, et al. The exomic landscape of t(14;18)-negative diffuse follicular lymphoma with 1p36 deletion. Br J Haematol 2018;180:391-4. [Crossref] [PubMed]
- Siddiqi IN, Friedman J, Barry-Holson KQ, et al. Characterization of a variant of t(14;18) negative nodal diffuse follicular lymphoma with CD23 expression, 1p36/TNFRSF14 abnormalities, and STAT6 mutations. Mod Pathol 2016;29:570-81. [Crossref] [PubMed]
- Dierlamm J, Michaux L, Wlodarska I, et al. Trisomy 3 in marginal zone B-cell lymphoma: a study based on cytogenetic analysis and fluorescence in situ hybridization. Br J Haematol 1996;93:242-9. [Crossref] [PubMed]
- Traverse-Glehen A, Felman P, Callet-Bauchu E, et al. A clinicopathological study of nodal marginal zone B-cell lymphoma. A report on 21 cases. Histopathology 2006;48:162-73. [Crossref] [PubMed]
- Louissaint A Jr, Schafernak KT, Geyer JT, et al. Pediatric-type nodal follicular lymphoma: a biologically distinct lymphoma with frequent MAPK pathway mutations. Blood 2016;128:1093-100. [Crossref] [PubMed]
- Schmidt J, Gong S, Marafioti T, et al. Genome-wide analysis of pediatric-type follicular lymphoma reveals low genetic complexity and recurrent alterations of TNFRSF14 gene. Blood 2016;128:1101-11. [Crossref] [PubMed]
- Louissaint A Jr, Ackerman AM, Dias-Santagata D, et al. Pediatric-type nodal follicular lymphoma: an indolent clonal proliferation in children and adults with high proliferation index and no BCL2 rearrangement. Blood 2012;120:2395-404. [Crossref] [PubMed]
- Lovisa F, Binatti A, Coppe A, et al. A high definition picture of key genes and pathways mutated in pediatric follicular lymphoma. Haematologica 2019;104:e406-9. [Crossref] [PubMed]
- Martin-Guerrero I, Salaverria I, Burkhardt B, et al. Recurrent loss of heterozygosity in 1p36 associated with TNFRSF14 mutations in IRF4 translocation negative pediatric follicular lymphomas. Haematologica 2013;98:1237-41. [Crossref] [PubMed]
- Ozawa MG, Bhaduri A, Chisholm KM, et al. A study of the mutational landscape of pediatric-type follicular lymphoma and pediatric nodal marginal zone lymphoma. Mod Pathol 2016;29:1212-20. [Crossref] [PubMed]
- Finn LS, Viswanatha DS, Belasco JB, et al. Primary follicular lymphoma of the testis in childhood. Cancer 1999;85:1626-35. [Crossref] [PubMed]
- Bacon CM, Ye H, Diss TC, et al. Primary follicular lymphoma of the testis and epididymis in adults. Am J Surg Pathol 2007;31:1050-8. [Crossref] [PubMed]
- Heller KN, Teruya-Feldstein J, La Quaglia MP, et al. Primary follicular lymphoma of the testis: excellent outcome following surgical resection without adjuvant chemotherapy. J Pediatr Hematol Oncol 2004;26:104-7. [Crossref] [PubMed]
- Lones MA, Raphael M, McCarthy K, et al. Primary follicular lymphoma of the testis in children and adolescents. J Pediatr Hematol Oncol 2012;34:68-71. [Crossref] [PubMed]
- Goodlad JR, Krajewski AS, Batstone PJ, et al. Primary cutaneous follicular lymphoma: a clinicopathologic and molecular study of 16 cases in support of a distinct entity. Am J Surg Pathol 2002;26:733-41. [Crossref] [PubMed]
- Zinzani PL, Quaglino P, Pimpinelli N, et al. Prognostic factors in primary cutaneous B-cell lymphoma: the Italian Study Group for Cutaneous Lymphomas. J Clin Oncol 2006;24:1376-82. [Crossref] [PubMed]
- Bergman R, Kurtin PJ, Gibson LE, et al. Clinicopathologic, immunophenotypic, and molecular characterization of primary cutaneous follicular B-cell lymphoma. Arch Dermatol 2001;137:432-9. [PubMed]
- Mirza I, Macpherson N, Paproski S, et al. Primary cutaneous follicular lymphoma: an assessment of clinical, histopathologic, immunophenotypic, and molecular features. J Clin Oncol 2002;20:647-55. [Crossref] [PubMed]
- Gángó A, Batai B, Varga M, et al. Concomitant 1p36 deletion and TNFRSF14 mutations in primary cutaneous follicle center lymphoma frequently expressing high levels of EZH2 protein. Virchows Arch 2018;473:453-62. [Crossref] [PubMed]
- Hallermann C, Kaune KM, Gesk S, et al. Molecular cytogenetic analysis of chromosomal breakpoints in the IGH, MYC, BCL6, and MALT1 gene loci in primary cutaneous B-cell lymphomas. J Invest Dermatol 2004;123:213-9. [Crossref] [PubMed]
- Dijkman R, Tensen CP, Jordanova ES, et al. Array-based comparative genomic hybridization analysis reveals recurrent chromosomal alterations and prognostic parameters in primary cutaneous large B-cell lymphoma. J Clin Oncol 2006;24:296-305. [Crossref] [PubMed]
- Barasch NJK, Liu YC, Ho J, et al. The molecular landscape and other distinctive features of primary cutaneous follicle center lymphoma. Hum Pathol 2020;106:93-105. [Crossref] [PubMed]
- Zhou XA, Yang J, Ringbloom KG, et al. Genomic landscape of cutaneous follicular lymphomas reveals 2 subgroups with clinically predictive molecular features. Blood Adv 2021;5:649-61. [Crossref] [PubMed]
Cite this article as: Zhou T, Pittaluga S, Jaffe ES. Molecular insights into the pathogenesis of follicular lymphoma. Ann Lymphoma 2021;5:12.