Tumor microenvironment of follicular lymphoma
Introduction
Follicular lymphoma (FL) is the most common indolent non-Hodgkin lymphoma and develops from precursor B cells in the germinal centre (GC) of lymphoid tissues (1,2). Understanding the pathogenesis of FL is critical to optimize therapeutic interventions and build upon prognostic models. A key component of FL pathogenesis is its microenvironment, which is the cellular and molecular environment in which the tumor resides, and includes immune cells, stromal cells, blood vessels and the extra-cellular matrix (3,4). Specific genetic aberrations within the tumor act to re-educate these neighbouring cells and there is ultimately an essential dependence on the microenvironment for tumor cell survival and proliferation (4). In this way, the FL tumor microenvironment is a critical mediator of lymphomagenesis with a fine balance between the promotion of a tumor permissive immune niche and suppression of antitumoral immune surveillance.
It is established that the FL immune milieu is a major contributor to patient outcome. Dave et al. first demonstrated that features of the non-malignant cells of the immune microenvironment correlated better with patient prognosis than the gene expression within the FL tumor cells themselves (5). An intratumoral T cell gene signature was associated with increased survival in FL whereas genes expressed by macrophages and follicular dendritic cells were associated with inferior outcomes (5). In subsequent work, the magnitude of intratumoral immune infiltration in FL was shown to form an independent positive prognostic marker in the rituximab era and identified the lymphoma microenvironment as a key determinant of early treatment failure (6). There was distinct clustering of immune molecules regardless of their categorization as immune effector, checkpoint or macrophage molecules, and having a low overall immune infiltrative state predicted for early progression of disease (6).
Recent technological advances have allowed for a more in-depth analysis of the FL microenvironment and revealed the marked heterogeneity of intratumoral immune cells. Further insights into this heterogeneity and complexity of the microenvironment is likely to offer new possibilities for patient risk stratification and therapeutic intervention.
To enable an understanding of the contribution of the FL microenvironment to disease, we start by characterizing the cellular components that comprise the FL microenvironment and the complex interplay of these cells are summarized. Next, key genetic aberrations that influences the FL microenvironment are explored. We then focus on the contribution of important non-cellular components, such as the cytokine milieu that further facilitate pathology via disturbances in homeostasis. Finally, we discuss the novel therapies that exploit pathways and mechanisms of the FL microenvironment to halt and or reverse disease progression.
Composition of the tumor microenvironment
The composition of the FL microenvironment is partially reflective of the physiological GC that it effaces, with neoplastic cells invading existing lymphoid follicles. By re-educating neighbouring microenvironmental cells toward a pro-tumoral state, FL tumor cells become highly dependent on them to survive and proliferate (4). The following section reviews the key cellular components of the FL microenvironment and includes immunocompetent lymphoid cells, macrophages and stromal cells, and should be read in reference to Figure 1.
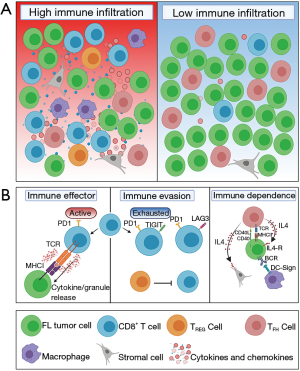
Cytotoxic CD8+ T cells
Tumor recognition by cytotoxic CD8+ T cells is a requirement for T cell mediated tumor control and may explain the spontaneous regressions that have been reported in FL (7). The FL microenvironment contains variable numbers of CD8+ T cells, but they are consistently lower than their CD4+ T cell counterpart, and are predominantly localised to peri-follicular regions (8,9). The immunological synapse between cytotoxic T cells and FL tumor cells has been demonstrated by confocal microscopy and is suggestive of tumor-specific antigen recognition (8). Upon activation, CD8+ T cells mediate their antitumoral function by cytokine production and granule release.
Identification of CD8+ T cells has mostly relied on immunohistochemistry (IHC), and to a lesser extent, flow cytometry. These studies have consistently shown favourable outcomes in patients with increased numbers of infiltrating CD8+ T cells (8,10-12) and more recently, transcriptional upregulation of T effector genes was shown to correlate with chemoimmunotherapy response in FL (13).Intriguingly, patients with an inflamed T effector phenotype had high tumor mutational loads (which may represent a proxy for neoantigen burden) compared to their non-inflamed counterparts (13). High throughput profiling techniques such as T cell receptor (TCR) repertoire sequencing has also allowed the field to move beyond relatively crude IHC studies to profile CD8+ T cells at considerable depth. TCR repertoire sequencing demonstrated clonal T cell expansions amongst high immune infiltrated FL subsets (6), with more detailed analysis of sorted T cell subsets revealing that these large clonal expansions resided within the cytotoxic T cell compartment (Nath et al., Blood Advances, in press). Further characterization of the neo-antigenic targets that these clones recognize is required.
The intratumoral CD8+ T cell population can be functionally subdivided into active, resting or exhausted (dysfunctional) states. Surprisingly, the high cell surface expression of the inhibitory receptor PD-1 (programmed cell death protein-1) is associated with an activated T cell state in FL, and it is the co-expression of both PD-1 and LAG3 (lymphocyte activation gene 3 protein) that define dysfunctional T cells with reduced capacity to perform classic effector functions (14). Increased PD-1/LAG3 co-expressing T cells adversely affected patient outcomes in a small series, and pending validation, warrant consideration as a predictive biomarker (14). Of note, these findings may explain the minimal response to anti-PD-1 therapy in FL (15) and a more suitable approach may be dual checkpoint blockade of both PD-1 and LAG3 to reinvigorate the exhausted CD8+ T cell subset. More recently, co-expression of the coinhibitory receptors TIGIT (T cell immunoglobulin and ITIM domain) and PD-1 was also shown to indicate an exhausted T cell phenotype, suggesting a role for TIGIT and PD-1 co-blockade (16). The factors (tumor and microenvironmental) driving the functional heterogeneity of intratumoral CD8+ T cells in FL requires further investigation.
Kiaii and colleagues demonstrated a marked dysregulation in the gene expression of highly purified CD8+ (and CD4+) intratumoral T cells in FL compared to healthy controls (17). One such example was the downregulation of ACTN1, which is involved in actin-based motility and cytoskeletal signalling, and contributed toward an impairment in the motility of FL tumor infiltrating lymphocytes (17). Interestingly, the coculture of healthy T cells with FL tumor cells induced similar defects in T cell protein expression (17). Collectively, these findings are strongly suggestive that FL tumor cells evade the immune microenvironment by directly altering the gene expression of neighbouring CD8+ and CD4+ T cells.
CD4+ T Cell populations
The dependency of FL tumor cells on surrounding CD4+ T cells to survive and expand has been demonstrated in patient-derived xenograft and in vitro culture models (18,19). Recent in-depth analysis using mass cytometry established the marked heterogeneity within the intratumoral CD4+ T cell population with the identification of at least 12 distinct subsets (20). In this study, the lack of co-stimulatory receptors (CD27 and CD28) on CD4+ T cells (consistent with a suppressive microenvironment) predicted for inferior outcomes, whereas the prognostic implications of PD-1 immune checkpoint expression varied between distinct subpopulations (20). In addition to the heterogeneity, the topographical distribution of CD4+ T cells may also play an independent role in prognostication and warrants integration into prognostic scores to improve risk stratification (21,22).
The following section describes the most well described CD4+ T cell subsets in FL and includes T Follicular Helper (TFH) cells, T Regulatory (TREG) cells and T Follicular Regulatory (TFR) cells.
T follicular helper cells
Under normal immune reactions T follicular helper (TFH) cells interact with antigen-activated GC B cells within the light zone of lymphoid follicles to support GC B cell selection and differentiation (23). GC TFH cells are characterized by a high expression of PD-1, ICOS and CXCR5, which is responsible for their follicular localization (24).
In FL, the malignant B cell retains its dependency on TFH cells and re-educates them towards a creating a tumor permissive immune niche. FL tumors are enriched with TFH cells and these highly express CD40L and IL4 compared to TFH cells from healthy nodes (25). The CD40L+ TFH cells interact with CD40+ neoplastic B cells, and together with the secretion of IL4 and IL21, appear to support tumor cell growth and survival (26). IL4 has further downstream effects including skewing of tumor associated macrophages (TAMs) towards cancer promotion (27) and secretion of CXCL12 by stromal cells to increase FL tumor cell recruitment and migration (28). It has also been demonstrated that both CD40L and IL4 have direct anti-apoptotic activity on FL B cells (25). Taken together, these observations suggest that FL tumor cells subvert the TFH population to their own advantage and a high TFH infiltrate has been shown to translate to inferior clinical outcomes (29).
T regulatory cells
FOXP3+CD4+CD25+ T Regulatory (TREG) cells are actively recruited and expanded within the FL microenvironment and FL tumor cells are able to convert conventional CD4+ T cells into CD4+ TREG cells (30-32). Yang and colleagues have shown that intratumoral FL TREG cells suppress the proliferation and cytokine production of neighbouring tumor infiltrating helper and cytotoxic T cells (33,34). Indeed, the diversity of the TREG TCR repertoire inversely correlated with intratumoral CD8+ TCR diversity in a small series, raising speculation that antigen-specific TREG cells suppress neighbouring FL-specific cytotoxic T cells (9).
A more comprehensive understanding of TREG cells is now possible with the emergence of mass cytometry. This has demonstrated that FL TREG cells are indeed a heterogenous population. PD-1 expression discriminated an activated subset (also co-expressing the activation-related molecules CD27, CD28, CD69, TIGIT) of intratumoral TREG cells (20). The increased PD-1 expression on FL TREG cells (in contrast to the Hodgkin lymphoma microenvironment where TREG cells are polarized toward a CXCR3+Tbet+ T helper-1 phenotype) has also been described by other groups (35). TIGIT expression on TREG cells in FL has also been shown to further enhance their suppressive properties (36).
As the TREG population appears to be driven at least in part by FL tumor cells, characteristics of this cell population deserves consideration as potential predictive biomarkers. In previous work, a high number of intra-tumoral TREG cells was surprisingly reported to be predictive of a favourable response to various chemotherapy regimens and one explanation for this may be direct TREG cell suppression of the tumor cells (37). However, in a more recent and uniformly treated FL cohort, the pre-treatment presence of high follicular or perifollicular TREG cells was associated with an inferior outcome (38). Moreover, the total TREG cell count had no impact on outcome (38). de Jong et al. showed that the density of TREG cells had opposite effects depending on what chemotherapy patients received, with a negative impact seen with fludarabine treated patients and a good prognostic impact reported with CVP (39). A simple explanation for these discrepancies may be the methodological differences between studies, which includes variations in treatment regimens. Indeed, the prognostic impact of FL TREG cells is circumvented with the addition of rituximab (40), suggesting that treatment may be the prime determinant for prognostic implications of TREG cells. Conceptually, the heterogeneity within TREG cells along with its differentiation state into the TFR subset might also be crucial to understanding its role as a predictive biomarker. Strategies that can recognize the TREG population at greater depth and studying its prognostic effects in a uniformly treated cohort in the rituximab era are required.
T follicular regulatory cells
Another T cell subset important to FL tumor cell growth and survival is the TFR cell. TFR cells make up a minor component of the FL immune microenvironment (~3% of CD4+ T cells) (25), and have functional similarities to both TFH and TREG cells. Though the precise role of FL TFR cells remains unclear, these FOXP3/CXCR5/ICOS co-expressing T cells are located within neoplastic follicles and thought to regulate TFH and B cell interactions, ultimately maintaining GC B cell proliferation (41). Though speculative, FL TFR cells may help explain the finding that a follicular distribution of FOXP3 T cells was associated with inferior clinical outcomes in the pre-rituximab era (38).
Natural killer (NK) cells
Although intratumoral NK cells only contribute toward a small percentage of the non-malignant cells in the FL microenvironment, the mature and cytotoxically active (CD56dim) NK cell subset is enriched in FL compared to healthy controls (42). This is relevant given the routine incorporation of rituximab into FL treatment algorithms and the significant role of NK cells in mediating rituximab’s antibody-dependent-cell-mediated cytotoxic (ADCC) activity against FL tumor cells (43). Using sequential FL tissue samples, it has been shown that rituximab induces a rapid and proliferative NK cell response (42), which may be attributed to the role of NK cells as key effectors of ADCC activity.
Given as ADCC tumor-cell killing is likely dependent on effector to target cell ratios, NK cell counts can be considered a potential biomarker in predicting response to therapeutic monoclonal antibodies. This was confirmed in a post-hoc analysis of the phase III GALLIUM trial where a low baseline peripheral blood NK cell count in FL patients treated with rituximab or obinutuzumab plus chemotherapy was independently associated with inferior survival outcomes (44).
Tumor-associated macrophages
Tissues macrophages are the end cells of the mononuclear phagocytic lineage and are derived from circulating monocytes that originate in the bone marrow (45). In a somewhat simplistic binary model, such macrophages can be further categorized based on their inflammatory states into antitumoral M1 (activated) or pro-tumoral M2 (alternatively activated) subtypes (46). It has been shown that an over-expression CCL2 (secreted by stromal cells) and IL4 in the FL microenvironment contributes toward monocyte recruitment and their subsequent polarization into M2 tumor-associated macrophages (TAMs) (47,48). FL TAMs favor malignant B cell growth via the downstream effects of dendritic cell-specific ICAM-3-grabbing non-integrin (DC-SIGN) engagement with mannosylated regions on the FL B cell receptor (48), and also through their angiogenic and immunosuppressive properties (49,50).
Perhaps not surprisingly, a high expression of FL TAMs was originally identified to indicate a poor prognosis (5,51). However, this initial observation has conflicted with more recent studies that incorporated rituximab into FL treatment regimens (52,53). A possible explanation is that TAMs act as effector cells in rituximab’s ADCC activity against FL tumor cells (43,54). Kridel and colleagues highlighted that prognostication by macrophage infiltration is dependent on patient treatment by showing that M2 macrophages favourably modulated the efficacy of rituximab in those who received doxorubicin-containing chemotherapy (55). Indeed, it has been previously demonstrated that the efficacy of doxorubicin is enhanced by macrophage activation (56) and that the ADCC activity of rituximab is more profound in macrophages polarized toward a M2 phenotype (57). And in support of this, a low M2 macrophage infiltration was associated with early treatment failure in a recent, comprehensive investigation of uniformly immunochemotherapy-treated FL patients (58).
Mesenchymal stromal cells (MSCs)
Within secondary lymphoid organs, there is a heterogenous population of non-haematopoietic cells of stromal origin collectively known as MSCs. FL tumor cells require MSCs for their in vitro expansion (59) and stromal cell generated cytokines such as CXCL12 has been shown to cause FL B cell activation, migration and adhesion (28,60).
MSCs can be further distinguished based on their morphology and function into subtypes that include fibroblastic reticular cells (FRCs) and follicular dendritic cells (FDCs). FL tumor cells upregulate the differentiation of MSCs into FRCs, which in turn support malignant cell survival via an immune escape phenomenon (61-63). Under normal conditions, FDCs present native antigens to B cells and contribute towards an efficient GC response (64). In FL, FDCs provide co-stimulatory signals that promote angiogenesis, cell adhesion, migration and ultimately favour lymphoma growth and dissemination (65). FDCs have been shown to communicate with neoplastic B cells via their expression of B cell activating factor (BAFF), and this appears to have an anti-apoptotic effect on FL tumor cells (66). It has also been recently shown that the EZH2 gain-of-function mutation in FL (~25–30% FL patients) reprograms the GC to generate an increased FDC-dependent aberrant proliferation of B cells (67).
Non-cellular components of the tumor microenvironment
Cytokines and chemokines are signalling proteins used by cells for chemotaxis and modulation of responses such as inflammation. Collectively termed the “cytokine milieu”, this milieu underlies the complex crosstalk of the various cells of the TME. FL cells both modulate and are modulated by the cytokine milieu, establishing a tumor promoting niche that is both immune privileged and growth factor rich. The cytokine milieu within the TME in FL is widely altered, and cytokine modulation has been advocated in FL as a therapeutic intervention. Most notably, interferon-alpha administered alone or in combination with chemotherapy has been shown to have anti-FL activity, but is also a cause of clinically significant adverse effects (68). A key lesson is that cytokines have pleiotropic effects within different immune cellular contexts, meaning that a highly targeted and sophisticated approach is required before the beneficial effects of cytokine modulation can be fully realised.
Interleukin-2 (IL2)
In vitro, IL2 or IL2-soluble IL2R (sIL2R) complexes diverts the maturation of CD4+ T cells towards the immunosuppressive TREG subtype (69), which may explain the observation in several studies that high levels of sIL2R is associated with progression and transformation in FL (69-72). Hence, IL2-receptor inhibition is currently being investigated as a therapeutic option in FL (73,74).
Interleukin-4 (IL4) and CXCL12
IL4 and CXCL12 are overexpressed in the FL TME, including TFH and stromal cells respectively (28). Experimentally, only IL4 high FL- TFH cells increased CXCL12 expression in committed stromal cells. Bearing this in mind, CXCL12 and its receptors CXCR4/CXCR7 have emerged as a key mechanism which allows communication between cancer and cells in the TME (75). CXCL12 directly promotes cell growth, metastasis and angiogenesis, whilst attracting even more CXCR4 bearing and CXCL12 producing cells, such as the aforementioned stromal cells to the tumor site, in an amplifying loop (75). Supporting this mechanism in FL, decreased levels of CXCR4 were associated with good prognosis in NHL (76).
Interleukin-12 (IL12)
Pre-clinically, IL12 was shown to have anti-tumor effects (77). However, a phase II clinical trial showed that a combination of IL12 and rituximab had reduced responses when compared to rituximab alone in NHLs (77). To determine the cause of experimental treatment failure, Yang et al. showed that in vitro exposure of CD4+ T cells to IL12 induced an exhausted phenotype via increased TIM-3expression (78). IL12 expression was confirmed in FL samples. Building on this, elevated CXCL9, a downstream inflammatory chemokine of IL12 signalling, was associated with poor outcomes in FL (79). A lymphomagenesis mechanism involving CXCL9 has been described in the associated disease diffuse large B-cell lymphoma (DLBCL) (80). These lines of evidence show that more experimental work should be done to illicit the underlying mechanisms of the IL12/TIM3/CXCL19 pathway and uncover a potential therapeutic target.
Prognostic value of serum cytokines
Serum cytokine levels have been linked to prognostication in FL, suggesting that a clinically validated serum-based test might have potential utility as a functional minimally invasive sequential biomarker (79). Eight cytokines (IL-1Ra, IL-6, IL-7, IL-10, IL-13, TNF-α, VEGF, and PDGF) were elevated in FL with high levels of TGF-β associated with superior overall survival and high levels of VEGF associated with poor progression free survival independent of lactate dehydrogenase (81). TGF-β has been variously described as having inhibitory roles in B-cell maturation whilst inducing exhaustion of effector memory T cells in NHLs (82,83). Finally, VEGF expression is upregulated and correlated to poor prognosis in FL as it mediates angiogenesis by stimulating the growth of vascular endothelial cells (84).
Genetic aberrations as determinants of the immune microenvironment
Several mutations in DNA repair, methylation and cell cycle control steps have been identified in FL. The t(14;18) (q32;q21) (IGH/BCL2) translocation, first reported in 1988, underlies over 90% of FL cases and is thought to be a founding genetic event (85). Unlike other oncogenes known to that point, BCL-2 did not regulate proliferation but rather it regulated apoptosis. The translocation of BCL2 to the promoter region of IGH causes overexpression of the anti-apoptotic protein BCL2. However, this translocation alone is insufficient to trigger lymphomagenesis as approximately 70% of healthy individuals bear this translocation without ever developing FL (86).
Besides genetic aberrations increasing the intrinsic fitness of the FL cell, the FL cell has been shown to influence and modulate the TME (4). This section focuses on the key mechanisms behind the genetic aberrations that allows FL to modulate and influence the TME (summarized in Figure 2). Of the large catalogue of mutations, the chromatin modifying genes (CMGs), EZH2 and CREBBP are the most commonly mutated genes in FL which have been implicated in TME modulation (87).
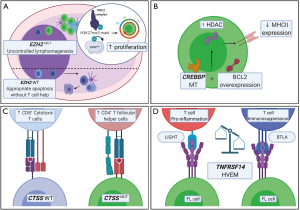
EZH2
EZH2 catalyses trimethylation of the polycomb repressive complex 2 which has downstream effects on transcriptional silencing (87,88). In FL, gain-of-function mutations of EZH2 recurrently occur at three sites, Y646 (also known as EZH2Y641F), A682 and A692 in approximately 25% of FLs with variant allele frequencies ranging from 2–61% (89-92). Mutations affecting Tyr 646 (Y646) in the SET domain of EZH2 increases the affinity for methylating the histone mark, H3K27me2 to H3K27me3 compared to wild-type protein (67). A copy of a wild-type EZH2 and mutant Y646 is required for lymphomagenesis as the wild-type enzyme favours the mono- and di- methylation steps, whilst the mutant enzyme is only hyperactive in the third trimethylation step (92). These gain-of-function mutations in EZH2 result in the perturbation of another gene, SESTRIN1, leading to downstream disruption of P53 mediated control over the mammalian target of rapamycin complex 1 (mTORC1) (93), allowing B cells to proliferate and accumulate.
Recently, EZH2 mutations were shown to initiate lymphomagenesis by reprograming the immune response (94). Normally, as part of the GC reaction, GC B-cells requires a TFH cell of the correct affinity to prevent apoptosis, whilst in EZH2 bearing mutants the apoptotic signal is evaded (95). When a CD40 blocking ligand is administered to inhibit the activity of TFH, the GC reaction was impaired in wild-type cells (67). However, no such impairment of the GC reaction was seen in EZH2 bearing mutants. In contrast, when lymphotoxin β was administered to block FDC activity, EZH2MUT cells had reduced fitness compared to wild-type cells. Taken together, this study shows how FL cells bearing EZH2Y641F mutations evades normal physiological control and requires an altered TME in the form of FDCs. Hence, inhibiting EZH2 activity is a promising potential therapy for FL (96).
MHC-I/II-deficient tumors, characterized by reduced levels of tumor-infiltrating lymphocytes, demonstrate a strong enrichment of EZH2 mutations (97). Importantly, EZH2 inhibitors restored MHC expression in EZH2-mutated human lymphoma cell-lines, suggesting a role for the way for development of complementary therapeutic approaches combining immunotherapy with epigenetic reprogramming.
CREBBP/EP300
CREBBP is another recurrently mutated CMG that modulates the TME in FL. CREBBP encodes a lysine acetyltransferase (KAT) which acetylates histone 3 and enables access to DNA by transcription factors (98). EP300 is an associated homolog of CREBBP with over 90% sequence homology and is thought to be functionally similar to CREBBP. Mutations in EP300 occur in approximately 20% of FL whereas CREBBP is mutated in 41–65% with either truncating mutations or inactivating mutations in the HAT domain that are functionally more severe (99,100).
The Stanford group demonstrated that mutations in CREBBP was associated with reduced MHC-II gene expression by microarray and flow-cytometry (98). Reduced MHC-II expression is associated with lymphomagenesis by interfering with the ability of T cells to detect aberrant cancer specific antigens (101). The normal function of CREBBP is antagonistic to that of the B-cell lymphoma 6 protein (BCL6)-Histone Deacetylase 3 (HDAC3) complexes (100,102). With CREBBP loss-of-function mutations, the deacetylation action of BCL6-HDAC3 complexes is perturbed but may be restored by inhibiting the action of HDAC3 (102). Building on these findings, Mondello et al. demonstrated that administration of HDAC3 inhibitors restored immune surveillance by the induction of interferon pathway and MHC-II genes (103).
CTSS
Besides CMGs, other genes also have been shown to be associated with TME modulation. One of these is CTSS that encodes for the lysosomal cysteine proteolytic enzyme Cathepsin S which has critical roles in MHC-II antigen presentation and GC maturation (104,105). Recently, two independent studies described recurrent CTSSY132 gain-of-function mutations in FL and determined its subsequent impact on the TME in FL. They demonstrated that CTSS is overexpressed and hyperactive in FL (106,107). This leads to enhanced MHC-II antigen presentation which promotes tumor growth by increasing the CD4+ TFH: cytotoxic CD8+ T cell ratio (106,107). Conversely, when CTSS activity is inhibited, communication between the FL cell and CD4+ TFH cells is reduced whilst CD8+ cytotoxic T cells increased their infiltration of the TME (106). In addition, CTSSY132 was associated with favourable outcomes with R-CHOP chemoimmunotherapy that plausibly supressed the CD4+ TFH cell enriched TME (107). CTSS inhibitors are a potential novel therapeutic strategy that would be particularly applicable for CTSS-hyperactive FL.
TNFRSF14
Another gene involved in FL TME modulation is TNFRSF14 which encodes for the herpes virus entry mediator (HVEM). Originally, binding of herpes simplex viral envelope glycoprotein D to this receptor was shown to be part of the viral entry mechanism. Two major families of ligands for HVEM may either activate or supress T-cell activity (108). The ligands belonging to the TNF-related cytokine family: lymphotoxin-like inducible protein that competes with glycoprotein D for herpes virus entry on T cells (LIGHT), and lymphotoxin-α are both proinflammatory (108). Conversely, ligands from the immunoglobulin related membrane protein family, B and T lymphocyte attenuator (BTLA) and CD160 supresses T-cell activity (109); 28–67% of FLs had recurrent alterations in the 1p36.32 region of TNFRSF14 and are associated with poor prognosis (110,111). Loss-of-function TNFRSF14 mutated lymphoma B cells induced a tumor supportive environment, with increased stromal activation and increased TFH cell recruitment due to disruption of inhibitory cell-cell interactions between the HVEM and BTLA (112). In elegant experiments, these investigators restored tumor suppression by delivery of soluble HVEM via gene modified CD19+ chimeric antigen receptor (CAR)-T cells (112). Conversely, mutations resulting in a loss of TNFRSF14 expression increased recognition of lymphoma B-cells by allogenic stem cell transplanted T cells resulting in increased eradication of lymphoma cells but may also result in undesirable graft versus host disease (113). In agreement, high expression of TNFRSF14 and low BTLA was found to be associated with poor prognosis in FL (114). The complex bi-directional signalling nature of TNFRSF14 clearly modulates the FL TME and awaits further characterization.
Novel therapies targeting the tumor microenvironment
The FL microenvironment can be therapeutically modulated to repress immune ‘ignorance’ and ‘evasion’ phenomena. Indeed, a high immune infiltrative state in FL predicted for improved responses to chemo-immunotherapy (6). As such, and with an improved understanding of the complex biology of the microenvironment, there is ongoing clinical development of an expanding number of immunotherapeutic targets in FL beyond anti-CD20 antibodies (Table 1), with several clinical trials demonstrating promising results. However, longer follow-up is required to determine treatment durability. There remains an ongoing need to determine predictive biomarkers of sensitivity to such agents and these biomarkers are likely to be treatment specific. The following section reviews the mechanistic evidence of novel therapies that directly or indirectly target the FL microenvironment. A more detailed review of novel immunotherapeutic agents is included elsewhere in this series by Khurana et al. entitled “Novel immunotherapy for FL”.
Table 1
Class | Agent | Phase [Estimated Enrolment] | Population and study design |
---|---|---|---|
ImmunomodulatoryAgents | CC-122 ( |
Ib [n=75] | R/R DLBCL, indolent NHL |
CC-122 + Obinutuzumab | |||
Lenalidomide ( |
III [n=840] | Front-line, high tumor burden FL | |
Post-induction PET positive patients randomized to anti-CD20 antibody maintenance +/- lenalidomide | |||
Anti-CD47 Antibodies | Hu5F9-G4 ( |
Ib/II [n=422] | R/R B-cell NHL |
Hu5F9-G4 (magrolimab) + Rituximab | |||
Preliminary data: ORR 71% (CR 43%) in FL, n=7 ( |
|||
BCL-2 Inhibitors | Venetoclax ( |
I/II [n=61] | Front-line, high tumor burden FL |
Lenalidomide + Venetoclax + Obinutuzumab | |||
Bispecific Antibodies | Blinatumomab ( |
I [n=44] | R/R B cell NHL |
Blinatumomab + Lenalidomide | |||
Glofitamab ( |
I [n=207] | R/R B cell NHL | |
Glofitamab + RO7227166 (CD19 targeted 41BB ligand), or obinutuzumab + RO7227166 | |||
Mosunetuzumab ( |
I [n=746] | R/R B cell NHL and CLL | |
Mosunetuzumab ± atezolizumab | |||
CAR Therapy | CAR NK cell ( |
I/II [n=36] | R/R B cell lymphoid malignancies |
Umbilical Cord Blood-Derived CAR NK Cells | |||
Preliminary data: ORR 73% (CR 64%), n=11 ( |
|||
CAR T cell ( |
II [n=160] | R/R indolent NHL | |
Axicabtagene Ciloleucel | |||
Preliminary data: ORR 94% (CR 80%) in FL, n=84 ( |
|||
Cancer Vaccines | NeoVax ( |
I [n=20] | Front-line FL |
Front-line rituximab followed by personalised neoantigen vaccine | |||
Oncoquest-L-Vaccine ( |
II [n=30] | Front-line, non-bulky FL | |
Autologous tumor-derived vaccine | |||
Tumour Vaccine ( |
I [n=20] | Relapsed FL | |
Personalized tumor vaccine + Nivolumab | |||
Immune CheckpointBlockade | Pembrolizumab ( |
I/II [n=134] | R/R Lymphomas |
Pembrolizumab + MK-4280 (anti-LAG3 antibody) | |||
Atezolizumab ( |
II [n=138] | R/R B cell NHL | |
Atezolizumab + Obinutuzumab + Venetoclax |
Abbreviations: R/R, relapsed/refractory; DLBCL, diffuse large B cell lymphoma; NHL, non-Hodgkin lymphoma; ORR, overall response rate; CR, complete response; n, number; FL, follicular lymphoma; CLL, chronic lymphocytic leukemia; NK, natural killer
Imunomodulatory agents
Lenalidomide is a second-generation immunomodulatory agent that targets the ubiquitously expressed E3 ubiquitin-ligase cereblon protein and promotes the degradation of haematopoietic transcription factors Aiolos and Ikaros (132). This has a multitude of downstream effects on immune cells within the tumor microenvironment. Ramsay and colleagues have shown that formation of the T cell immune synapse is impaired in FL, and that this defect can be restored with lenalidomide (133). Additional antitumoral effects of lenalidomide includes stimulation of the proliferative and functional capacity of effector T cells (134) and the invigoration of NK cell activity (135). Enhancing NK cell-mediated ADCC with lenalidomide may contribute toward a synergistic action with anti-CD20 monoclonal antibodies (136,137). Indeed, lenalidomide in combination with rituximab has shown to be efficacious in large phase 3 clinical trials in both de-novo and relapsed FL (138,139), and avadomide (CC-122), a novel immunomodulatory agent, is also being investigated in combination with rituximab in relapsed and refractory FL (140). The synergistic activity of immunomodulatory agents with other drug classes is being actively tested using novel doublet and triplet combinations and a major focus of such approaches should be to determine predictive microenvironmental markers. One such study revealed that combination therapy with lenalidomide and rituximab promoted the beneficial role of intratumoral GATA3+ T helper 2 cells, whilst counteracting the negative effect of PD-1+ T cells (141). These findings require validation in an independent cohort.
Anti-CD47 monoclonal antibody
CD47 is upregulated on malignant cell surfaces and interacts with its receptors on macrophages to evade phagocytosis (142). Preventing this anti-phagocytic signal can be very effective as not only would macrophage mediated tumor-phagocytosis increase, but it would also allow for phagocytic cross-presentation of tumor antigens to trigger an anti-tumor cytotoxic T cell response (143). Hu5F9-G4 is an immune macrophage checkpoint inhibitor blocking CD47 and has shown promising synergistic activity with rituximab in relapsed FL in an early phase clinical trial (118). A phase II study of this combination is currently accruing patients (ClinicalTrials.gov number, NCT02953509) (117). A growing body of evidence suggests that other macrophage immune checkpoints may also serve as potentially clinically actionable targets in lymphoma (144).
Immune checkpoint blockade
Cancer immunotherapy targeting the PD-1 ligand (PD-L1) axis can elicit dramatic responses in a subset of hematologic malignancies. A key marker of responsiveness to anti-PD-1 or anti-PD-L1 therapy is PD-L1 overexpression. This can be driven by gene amplification, as reported in Hodgkin lymphoma and primary mediastinal large B cell lymphoma (145), or structural variants disrupting the 3’ region of the PD-L1 gene, as reported in adult T cell leukemia and DLBCL (146). The absence of PD-L1 gene amplification or structural variants in FL may account for the modest clinical responses to single-agent PD-1 checkpoint blockade in this disease (15,147). However, combining the PD-1 inhibitor pembrolizumab with rituximab yielded promising results, with a high intratumoral CD8+ T effector score predicting for response in this small FL cohort (148). Dual-checkpoint blockade with anti-PD-1 and anti-LAG3 antibody therapy is also being actively investigated in FL (130), and such an approach may reinvigorate the exhausted intratumoral T cell population.
CAR T cell therapy and bispecific antibodies
CD19 directed autologous CAR T cell therapy is effective and approved in patients with histologic transformation of FL (tFL) (149,150), and promising results have been reported with allogenic anti-CD19 CAR NK cells (124). CAR T cell therapy is being actively investigated in relapsed and refractory FL with early experience demonstrating an over 80% complete response rate (151). Strategies to increase the potency of CAR T cells by enhancing the antitumoral components of the microenvironment are also being explored. Boice and colleagues developed an innovative approach in the pre-clinical setting where CAR T cells were used as ‘micro-pharmacies’ to deliver anti-cancer proteins and restore host tumor suppression (112). They showed that loss of the HVEM (TNFRSF14) receptor gene, which is commonly mutated in FL, leads to a tumor-supportive microenvironment by exacerbating recruitment of TFH cells and by activating the surrounding lymphoid stroma (112). Tumor suppression was successfully restored by administering a HVEM ectopic domain protein (soIHVEM) using modified CAR T cells that continually produced soIHVEM (112). Curran et al. enhanced the antitumoral effects of the microenvironment by modifying the CAR T cell construct to constitutively express CD40 ligand (152).
Mosunetuzumab, a T cell bispecific antibody (TCB) binding simultaneously to CD3 on cytotoxic T cells and CD20 on the malignant B cell results in the formation of an immunological synapse and subsequent T cell activation (153). Mosunetuzumab monotherapy showed modest clinical activity in heavily pre-treated FL patients in an early phase clinical trial, including a small subset with prior exposure to CAR T cell therapy (154). Another novel TCB with a ‘2:1’ configuration for increased tumor avidity (two fragment antigen binding regions for CD20, and one for CD3) is currently being investigated in relapsed FL (155).
Neoantigens, abscopal effect and immunogenic cell death
FL Neoantigens
Neoantigens are peptides generated from somatically acquired tumor genetic mutations that are then presented to the microenvironment in the context of major histocompatibility complexes (MHCs) (156). There is compelling evidence that the intratumoral T cell repertoire recognizes and elicits an immune response to epitopes that arise from cancer neoantigens. In FL, the malignant B lymphocytes undergo ongoing somatic hypermutation of V(D)J alleles of their immunoglobulin genes generating unique B cell receptor idiotypes (157). Khodadoust et al. used proteomics to demonstrate that it is these immunoglobulin-derived FL neoantigens that are presented by class II MHC to CD4+ T cells, whilst MHC presentation of non-immunoglobulin neoantigens was not seen (158). Nielsen and colleagues identified only small subsets of FL patients harbouring low frequency neoantigen-specific CD8+ T cells derived from mutant CREBBP and MEF2B genes (159). Interestingly, Bolen et al. used tumor mutational burden as a proxy for immunogenic neoantigen formation and showed that a high mutation load identified an ‘inflamed’ FL subset with a high effector T cell signature (13). A greater recognition of the immunogenic neoantigens in FL is critical to harness their anti-tumor effects via therapeutic manipulation with adoptive cell and vaccine therapy.
Abscopal effect and immunogenic cell death
The effect of local radiotherapy leading to regression of metastatic cancer at distant sites is known as the abscopal effect (160). The mechanism for this rare phenomenon is immune-mediated, whereby irradiation causes tumor cell apoptosis, and subsequent liberation of neoantigens (161). This increase in neoantigens can generate a cytotoxic tumor-specific immune response, resulting in both local and distant antitumor effects. In addition to this, tumor cell injury stimulates an immune response through the release of danger-associated molecular patterns (DAMPs), which in turn induces immunogenic cell death of malignant cells and acts as a catalyst to promote further immune activation (162) (Figure 3). Radiotherapy is used as part of the treatment armamentarium in FL. Although most studies of the abscopal effect have focused on solid tumors, FL has been shown to be the most common lymphoma subtype associated with abscopal regressions (163). Efforts to boost abscopal responses by combining radiotherapy with immunomodulation and thereby overcoming the suppressive features of the immune microenvironment need to be further explored in FL (164).
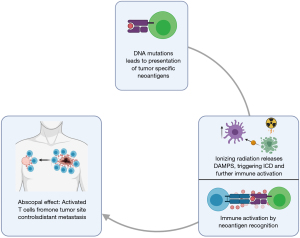
Conclusions
Immune evasion is a hallmark of cancer (165). Paradoxically, FL is a cancer of the immune system that originates from the GC of lymphoid tissues. Undoubtedly, developments in high-throughput genome sequencing, computer technology and transgenic animal models have led to unprecedented advances in our understanding of the genetic aberrations and down-stream signalling events that underpin the pathogenesis of the malignant B cell. However, our understanding of the bi-directional interplay between the FL B cell and the cellular, stromal and cytokine/chemokine components within which they reside remains at a relatively primitive stage. The field remains wide-open. Lack of cell-lines, relevant in vitro and humanised animal models, integrated biobanking and difficulty in obtaining sequential node biopsies during treatment remain obstacles to be overcome, if we are to fully harness the therapeutic potential of TME modulation.
Acknowledgments
All Figures were created with Biorender.com.
Funding: This work was supported by a Haematology Society of Australia and New Zealand and Leukaemia Foundation PhD scholarship (KN); a University of Queensland Research Training Scholarship (HT); and the Leukaemia Foundation, the Mater Foundation and the National Health and Medical Research Council (MKG).
Footnote
Provenance and Peer Review: This article was commissioned by the Guest Editors (Mark Roschewski, Carla Casulo) for the series “Follicular Lymphoma” published in Annals of Lymphoma. The article has undergone external peer review.
Conflicts of Interest: All authors have completed the ICMJE uniform disclosure form (available at http://dx.doi.org/10.21037/aol-20-55). MKG reports grants or contracts received from Janssen, BMS and Celgene, financial support received from Roche, Janssen, Merck, Amgen, Gilead, and has served on a board for Merck. The authors have no other conflicts of interest to declare.
Ethical Statement: The authors are accountable for all aspects of the work in ensuring that questions related to the accuracy or integrity of any part of the work are appropriately investigated and resolved.
Open Access Statement: This is an Open Access article distributed in accordance with the Creative Commons Attribution-NonCommercial-NoDeri 4.0 International License (CC BY-NC-ND 4.0), which permits the non-commercial replication and distribution of the article with the strict proviso that no changes or edits are made and the original work is properly cited (including links to both the formal publication through the relevant DOI and the license). See: https://creativecommons.org/licenses/by-nc-nd/4.0/.
References
- Teras LR, DeSantis CE, Cerhan JR, et al. 2016 US lymphoid malignancy statistics by World Health Organization subtypes. CA Cancer J Clin 2016;66:443-59. [Crossref] [PubMed]
- Carbone A, Roulland S, Gloghini A, et al. Follicular lymphoma. Nat Rev Dis Primers 2019;5:83. [Crossref] [PubMed]
- Fowler NH, Cheah CY, Gascoyne RD, et al. Role of the tumor microenvironment in mature B-cell lymphoid malignancies. Haematologica 2016;101:531-40. [Crossref] [PubMed]
- Scott DW, Gascoyne RD. The tumour microenvironment in B cell lymphomas. Nat Rev Cancer 2014;14:517-34. [Crossref] [PubMed]
- Dave SS, Wright G, Tan B, et al. Prediction of survival in follicular lymphoma based on molecular features of tumor-infiltrating immune cells. N Engl J Med 2004;351:2159-69. [Crossref] [PubMed]
- Tobin JWD, Keane C, Gunawardana J, et al. Progression of Disease Within 24 Months in Follicular Lymphoma Is Associated With Reduced Intratumoral Immune Infiltration. J Clin Oncol. 2019;37:3300-9. [Crossref] [PubMed]
- Horning SJ, Rosenberg SA. The natural history of initially untreated low-grade non-Hodgkin's lymphomas. N Engl J Med 1984;311:1471-5. [Crossref] [PubMed]
- Laurent C, Muller S, Do C, et al. Distribution, function, and prognostic value of cytotoxic T lymphocytes in follicular lymphoma: a 3-D tissue-imaging study. Blood 2011;118:5371-9. [Crossref] [PubMed]
- Liu X, Venkataraman G, Lin J, et al. Highly clonal regulatory T-cell population in follicular lymphoma - inverse correlation with the diversity of CD8+ T cells. Oncoimmunology 2015;4:e1002728 [Crossref] [PubMed]
- Alvaro T, Lejeune M, Salvado MT, et al. Immunohistochemical patterns of reactive microenvironment are associated with clinicobiologic behavior in follicular lymphoma patients. J Clin Oncol 2006;24:5350-7. [Crossref] [PubMed]
- Wahlin BE, Sander B, Christensson B, et al. CD8+ T-Cell Content in Diagnostic Lymph Nodes Measured by Flow Cytometry Is a Predictor of Survival in Follicular Lymphoma. Clin Cancer Res 2007;13:388. [Crossref] [PubMed]
- Wahlin BE, Aggarwal M, Montes-Moreno S, et al. A Unifying Microenvironment Model in Follicular Lymphoma: Outcome Is Predicted by Programmed Death-1–Positive, Regulatory, Cytotoxic, and Helper T Cells and Macrophages. Clin Cancer Res 2010;16:637. [Crossref] [PubMed]
- Bolen CR, McCord R, Huet S, et al. Mutation load and an effector T-cell gene signature may distinguish immunologically distinct and clinically relevant lymphoma subsets. Blood Adv 2017;1:1884-90. [Crossref] [PubMed]
- Yang ZZ, Kim HJ, Villasboas JC, et al. Expression of LAG-3 defines exhaustion of intratumoral PD-1(+) T cells and correlates with poor outcome in follicular lymphoma. Oncotarget 2017;8:61425-39. [Crossref] [PubMed]
- Armand P, Janssens A, Gritti G, et al. Efficacy and safety results from CheckMate 140, a phase 2 study of nivolumab for relapsed/refractory follicular lymphoma. Blood 2021;137:637-45. [Crossref] [PubMed]
- Josefsson SE, Beiske K, Blaker YN, et al. TIGIT and PD-1 Mark Intratumoral T Cells with Reduced Effector Function in B-cell Non-Hodgkin Lymphoma. Cancer Immunol Res 2019;7:355-62. [Crossref] [PubMed]
- Kiaii S, Clear AJ, Ramsay AG, et al. Follicular lymphoma cells induce changes in T-cell gene expression and function: potential impact on survival and risk of transformation. J Clin Oncol 2013;31:2654-61. [Crossref] [PubMed]
- Umetsu DT, Esserman L, Donlon TA, et al. Induction of proliferation of human follicular (B type) lymphoma cells by cognate interaction with CD4+ T cell clones. J Immunol 1990;144:2550. [PubMed]
- Burack WR, Spence JM, Spence JP, et al. Patient-derived xenografts of low-grade B-cell lymphomas demonstrate roles of the tumor microenvironment. Blood Adv 2017;1:1263-73. [Crossref] [PubMed]
- Yang ZZ, Kim HJ, Villasboas JC, et al. Mass Cytometry Analysis Reveals that Specific Intratumoral CD4+ T Cell Subsets Correlate with Patient Survival in Follicular Lymphoma. Cell Rep 2019;26:2178-93.e3. [Crossref] [PubMed]
- Mondello P, Fama A, Larson MC, et al. Intrafollicular CD4+ T-Cells As an Independent Predictor of Early Clinical Failure in Newly Diagnosed Follicular Lymphoma. Blood 2019;134:121. [Crossref]
- Mondello P, Fama A, Larson MC, et al. Prognostic relevance of CD4+ T-cells in the microenvironment of newly diagnosed follicular lymphoma (FL) patients is independent of the tumor gene expression profile. J Clin Oncol 2020;38:8052. [Crossref]
- Mlynarczyk C, Fontán L, Melnick A. Germinal center-derived lymphomas: The darkest side of humoral immunity. Immunol Rev 2019;288:214-39. [Crossref] [PubMed]
- Crotty S. T follicular helper cell differentiation, function, and roles in disease. Immunity 2014;41:529-42. [Crossref] [PubMed]
- Amé-Thomas P, Le Priol J, Yssel H, et al. Characterization of intratumoral follicular helper T cells in follicular lymphoma: role in the survival of malignant B cells. Leukemia 2012;26:1053-63. [Crossref] [PubMed]
- Wood B, Sikdar S, Choi SJ, et al. Abundant expression of interleukin-21 receptor in follicular lymphoma cells is associated with more aggressive disease. Leuk Lymphoma 2013;54:1212-20. [Crossref] [PubMed]
- Gocheva V, Wang HW, Gadea BB, et al. IL-4 induces cathepsin protease activity in tumor-associated macrophages to promote cancer growth and invasion. Genes Dev 2010;24:241-55. [Crossref] [PubMed]
- Pandey S, Mourcin F, Marchand T, et al. IL-4/CXCL12 loop is a key regulator of lymphoid stroma function in follicular lymphoma. Blood 2017;129:2507-18. [Crossref] [PubMed]
- Richendollar BG, Pohlman B, Elson P, et al. Follicular programmed death 1–positive lymphocytes in the tumor microenvironment are an independent prognostic factor in follicular lymphoma. Human Pathology 2011;42:552-7. [Crossref] [PubMed]
- Ai WZ, Hou JZ, Zeiser R, et al. Follicular lymphoma B cells induce the conversion of conventional CD4+ T cells to T-regulatory cells. Int J Cancer 2009;124:239-44. [Crossref] [PubMed]
- Yang ZZ, Novak AJ, Ziesmer SC, et al. CD70+ non-Hodgkin lymphoma B cells induce Foxp3 expression and regulatory function in intratumoral CD4+CD25 T cells. Blood 2007;110:2537-44. [Crossref] [PubMed]
- Le KS, Thibult ML, Just-Landi S, et al. Follicular B Lymphomas Generate Regulatory T Cells via the ICOS/ICOSL Pathway and Are Susceptible to Treatment by Anti-ICOS/ICOSL Therapy. Cancer Res 2016;76:4648. [Crossref] [PubMed]
- Yang ZZ, Novak AJ, Stenson MJ, et al. Intratumoral CD4+CD25+ regulatory T-cell-mediated suppression of infiltrating CD4+ T cells in B-cell non-Hodgkin lymphoma. Blood 2006;107:3639-46. [Crossref] [PubMed]
- Yang ZZ, Novak AJ, Ziesmer SC, et al. Attenuation of CD8(+) T-cell function by CD4(+)CD25(+) regulatory T cells in B-cell non-Hodgkin's lymphoma. Cancer Res 2006;66:10145-52. [Crossref] [PubMed]
- El Daker S, Gao Q, Roshal M, et al. CD4 Lymphocytes and T Regulatory Cells Show Distinct Phenotypes in Follicular Lymphoma and Classical Hodgkin Lymphoma Which May Account for Differences in Responses to Checkpoint Therapy. Blood 2019;134:1513. [Crossref]
- Yang ZZ, Kim HJ, Wu H, et al. TIGIT Expression Is Associated with T-cell Suppression and Exhaustion and Predicts Clinical Outcome and Anti-PD-1 Response in Follicular Lymphoma. Clin Cancer Res 2020;26:5217-31. [Crossref] [PubMed]
- Carreras J, Lopez-Guillermo A, Fox BC, et al. High numbers of tumor-infiltrating FOXP3-positive regulatory T cells are associated with improved overall survival in follicular lymphoma. Blood 2006;108:2957-64. [Crossref] [PubMed]
- Farinha P, Al-Tourah A, Gill K, et al. The architectural pattern of FOXP3-positive T cells in follicular lymphoma is an independent predictor of survival and histologic transformation. Blood 2010;115:289. [Crossref] [PubMed]
- de Jong D, Koster A, Hagenbeek A, et al. Impact of the tumor microenvironment on prognosis in follicular lymphoma is dependent on specific treatment protocols. Haematologica 2009;94:70-7. [Crossref] [PubMed]
- Xerri L, Huet S, Venstrom JM, et al. Rituximab treatment circumvents the prognostic impact of tumor-infiltrating T-cells in follicular lymphoma patients. Hum Pathol 2017;64:128-36. [Crossref] [PubMed]
- Xie MM, Dent AL. Unexpected Help: Follicular Regulatory T Cells in the Germinal Center. Front Immunol 2018;9:1536. [Crossref] [PubMed]
- Enqvist M, Jacobs B, Junlén HR, et al. Systemic and Intra-Nodal Activation of NK Cells After Rituximab Monotherapy for Follicular Lymphoma. Front Immunol 2019;10:2085. [Crossref] [PubMed]
- Cheson BD, Leonard JP. Monoclonal antibody therapy for B-cell non-Hodgkin's lymphoma. N Engl J Med 2008;359:613-26. [Crossref] [PubMed]
- Klanova M, Oestergaard MZ, Trněný M, et al. Prognostic Impact of Natural Killer Cell Count in Follicular Lymphoma and Diffuse Large B-cell Lymphoma Patients Treated with Immunochemotherapy. Clin Cancer Res 2019;25:4634-43. [Crossref] [PubMed]
- Wynn TA, Chawla A, Pollard JW. Macrophage biology in development, homeostasis and disease. Nature 2013;496:445-55. [Crossref] [PubMed]
- DeNardo DG, Ruffell B. Macrophages as regulators of tumour immunity and immunotherapy. Nat Rev Immunol 2019;19:369-82. [Crossref] [PubMed]
- Guilloton F, Caron G, Menard C, et al. Mesenchymal stromal cells orchestrate follicular lymphoma cell niche through the CCL2-dependent recruitment and polarization of monocytes. Blood 2012;119:2556-67. [Crossref] [PubMed]
- Amin R, Mourcin F, Uhel F, et al. DC-SIGN-expressing macrophages trigger activation of mannosylated IgM B-cell receptor in follicular lymphoma. Blood 2015;126:1911-20. [Crossref] [PubMed]
- Clear AJ, Lee AM, Calaminici M, et al. Increased angiogenic sprouting in poor prognosis FL is associated with elevated numbers of CD163+ macrophages within the immediate sprouting microenvironment. Blood 2010;115:5053-6. [Crossref] [PubMed]
- Carbonnelle-Puscian A, Copie-Bergman C, Baia M, et al. The novel immunosuppressive enzyme IL4I1 is expressed by neoplastic cells of several B-cell lymphomas and by tumor-associated macrophages. Leukemia 2009;23:952-60. [Crossref] [PubMed]
- Farinha P, Masoudi H, Skinnider BF, et al. Analysis of multiple biomarkers shows that lymphoma-associated macrophage (LAM) content is an independent predictor of survival in follicular lymphoma (FL). Blood 2005;106:2169-74. [Crossref] [PubMed]
- Taskinen M, Karjalainen-Lindsberg ML, Nyman H, et al. A high tumor-associated macrophage content predicts favorable outcome in follicular lymphoma patients treated with rituximab and cyclophosphamide-doxorubicin-vincristine-prednisone. Clin Cancer Res 2007;13:5784-9. [Crossref] [PubMed]
- Canioni D, Salles G, Mounier N, et al. High numbers of tumor-associated macrophages have an adverse prognostic value that can be circumvented by rituximab in patients with follicular lymphoma enrolled onto the GELA-GOELAMS FL-2000 trial. J Clin Oncol 2008;26:440-6. [Crossref] [PubMed]
- Maloney DG. Anti-CD20 antibody therapy for B-cell lymphomas. N Engl J Med 2012;366:2008-16. [Crossref] [PubMed]
- Kridel R, Xerri L, Gelas-Dore B, et al. The Prognostic Impact of CD163-Positive Macrophages in Follicular Lymphoma: A Study from the BC Cancer Agency and the Lymphoma Study Association. Clin Cancer Res 2015;21:3428-35. [Crossref] [PubMed]
- De Palma M, Lewis CE. Macrophage regulation of tumor responses to anticancer therapies. Cancer Cell 2013;23:277-86. [Crossref] [PubMed]
- Leidi M, Gotti E, Bologna L, et al. M2 Macrophages Phagocytose Rituximab-Opsonized Leukemic Targets More Efficiently than M1 Cells In Vitro. J Immunol 2009;182:4415. [Crossref] [PubMed]
- Stevens WBC, Mendeville M, Redd R, et al. Prognostic relevance of CD163 and CD8 combined with EZH2 and gain of chromosome 18 in follicular lymphoma: a study by the Lunenburg Lymphoma Biomarker Consortium. Haematologica 2017;102:1413-23. [Crossref] [PubMed]
- Weekes CD, Pirruccello SJ, Vose JM, et al. Lymphoma cells associated with bone marrow stromal cells in culture exhibit altered growth and survival. Leuk Lymphoma 1998;31:151-65. [Crossref] [PubMed]
- Husson H, Freedman AS, Cardoso AA, et al. CXCL13 (BCA-1) is produced by follicular lymphoma cells: role in the accumulation of malignant B cells. Br J Haematol 2002;119:492-5. [Crossref] [PubMed]
- Amé-Thomas P, Maby-El Hajjami H, Monvoisin C, et al. Human mesenchymal stem cells isolated from bone marrow and lymphoid organs support tumor B-cell growth: role of stromal cells in follicular lymphoma pathogenesis. Blood 2007;109:693-702. [Crossref] [PubMed]
- Maby-El Hajjami H, Amé-Thomas P, Pangault C, et al. Functional Alteration of the Lymphoma Stromal Cell Niche by the Cytokine Context: Role of Indoleamine-2,3 Dioxygenase. Cancer Res 2009;69:3228. [Crossref] [PubMed]
- Lukacs-Kornek V, Malhotra D, Fletcher AL, et al. Regulated release of nitric oxide by nonhematopoietic stroma controls expansion of the activated T cell pool in lymph nodes. Nat Immunol 2011;12:1096-104. [Crossref] [PubMed]
- Heesters BA, Myers RC, Carroll MC. Follicular dendritic cells: dynamic antigen libraries. Nat Rev Immunol 2014;14:495-504. [Crossref] [PubMed]
- Matas-Céspedes A, Rodriguez V, Kalko SG, et al. Disruption of Follicular Dendritic Cells–Follicular Lymphoma Cross-talk by the Pan-PI3K Inhibitor BKM120 (Buparlisib). Clin Cancer Res 2014;20:3458. [Crossref] [PubMed]
- Mourcin F, Pangault C, Amin-Ali R, et al. Stromal cell contribution to human follicular lymphoma pathogenesis. Front Immunol 2012;3:280. [Crossref] [PubMed]
- Béguelin W, Teater M, Meydan C, et al. Mutant EZH2 Induces a Pre-malignant Lymphoma Niche by Reprogramming the Immune Response. Cancer Cell 2020;37:655-73.e11. [Crossref] [PubMed]
- Baldo P, Rupolo M, Compagnoni A, et al. Interferon-alpha for maintenance of follicular lymphoma. Cochrane Database Syst Rev 2010;CD004629 [PubMed]
- Yang ZZ, Grote DM, Ziesmer SC, et al. Soluble IL-2Rα facilitates IL-2-mediated immune responses and predicts reduced survival in follicular B-cell non-Hodgkin lymphoma. Blood 2011;118:2809-20. [Crossref] [PubMed]
- Umino K, Fujiwara SI, Ito S, et al. Serum soluble interleukin-2 receptor level at diagnosis predicts transformation in patients with follicular lymphoma. Leuk Lymphoma 2017;58:316-23. [Crossref] [PubMed]
- Kusano Y, Yokoyama M, Terui Y, et al. High pretreatment level of soluble interleukin-2 receptor is a robust prognostic factor in patients with follicular lymphoma treated with R-CHOP-like therapy. Blood Cancer J 2017;7:e614 [Crossref] [PubMed]
- Binder M, O'Byrne MM, Maurer MJ, et al. Associations between elevated pre-treatment serum cytokines and peripheral blood cellular markers of immunosuppression in patients with lymphoma. Am J Hematol 2017;92:752-8. [Crossref] [PubMed]
- Price-Troska T, Yang ZZ, Diller D, et al. Inhibiting IL-2 signaling and the regulatory T-cell pathway using computationally designed peptides. Invest New Drugs 2019;37:9-16. [Crossref] [PubMed]
- Veeramani S, Blackwell SE, Thiel WH, et al. An RNA Aptamer-Based Biomarker Platform Demonstrates High Soluble CD25 Occupancy by IL2 in the Serum of Follicular Lymphoma Patients. Cancer Immunol Res 2019;7:1511-22. [Crossref] [PubMed]
- Guo F, Wang Y, Liu J, et al. CXCL12/CXCR4: a symbiotic bridge linking cancer cells and their stromal neighbors in oncogenic communication networks. Oncogene 2016;35:816-26. [Crossref] [PubMed]
- Mazur G, Butrym A, Kryczek I, et al. Decreased expression of CXCR4 chemokine receptor in bone marrow after chemotherapy in patients with non-Hodgkin lymphomas is a good prognostic factor. PLoS One 2014;9:e98194 [Crossref] [PubMed]
- Ansell SM, Geyer SM, Maurer MJ, et al. Randomized phase II study of interleukin-12 in combination with rituximab in previously treated non-Hodgkin's lymphoma patients. Clin Cancer Res 2006;12:6056-63. [Crossref] [PubMed]
- Yang ZZ, Grote DM, Ziesmer SC, et al. IL-12 upregulates TIM-3 expression and induces T cell exhaustion in patients with follicular B cell non-Hodgkin lymphoma. J Clin Invest 2012;122:1271-82. [Crossref] [PubMed]
- Mir MA, Maurer MJ, Ziesmer SC, et al. Elevated serum levels of IL-2R, IL-1RA, and CXCL9 are associated with a poor prognosis in follicular lymphoma. Blood 2015;125:992-8. [Crossref] [PubMed]
- Ruiduo C, Ying D, Qiwei W. CXCL9 promotes the progression of diffuse large B-cell lymphoma through up-regulating β-catenin. Biomed Pharmacother 2018;107:689-95. [Crossref] [PubMed]
- Labidi SI, Ménétrier-Caux C, Chabaud S, et al. Serum cytokines in follicular lymphoma. Correlation of TGF-β and VEGF with survival. Ann Hematol 2010;89:25-33. [Crossref] [PubMed]
- Yang ZZ, Grote DM, Xiu B, et al. TGF-β upregulates CD70 expression and induces exhaustion of effector memory T cells in B-cell non-Hodgkin's lymphoma. Leukemia 2014;28:1872-84. [Crossref] [PubMed]
- Lebman DA, Edmiston JS. The role of TGF-β in growth, differentiation, and maturation of B lymphocytes. Microbes Infect 1999;1:1297-304. [Crossref] [PubMed]
- Yang J, Li W, He X, et al. VEGF overexpression is a valuable prognostic factor for non-Hodgkin's lymphoma evidence from a systemic meta-analysis. Dis Markers 2015;2015:786790 [Crossref] [PubMed]
- Vaux DL, Cory S, Adams JM. Bcl-2 gene promotes haemopoietic cell survival and cooperates with c-myc to immortalize pre-B cells. Nature 1988;335:440-2. [Crossref] [PubMed]
- Liu Y, Hernandez AM, Shibata D, et al. BCL2 translocation frequency rises with age in humans. Proc Natl Acad Sci U S A 1994;91:8910-4. [Crossref] [PubMed]
- Green MR. Chromatin modifying gene mutations in follicular lymphoma. Blood 2018;131:595-604. [Crossref] [PubMed]
- Viré E, Brenner C, Deplus R, et al. The Polycomb group protein EZH2 directly controls DNA methylation. Nature 2006;439:871-4. [Crossref] [PubMed]
- Bödör C, Grossmann V, Popov N, et al. EZH2 mutations are frequent and represent an early event in follicular lymphoma. Blood 2013;122:3165-8. [Crossref] [PubMed]
- Huet S, Xerri L, Tesson B, et al. EZH2 alterations in follicular lymphoma: biological and clinical correlations. Blood Cancer J 2017;7:e555 [Crossref] [PubMed]
- Morin RD, Mendez-Lago M, Mungall AJ, et al. Frequent mutation of histone-modifying genes in non-Hodgkin lymphoma. Nature 2011;476:298-303. [Crossref] [PubMed]
- Sneeringer CJ, Scott MP, Kuntz KW, et al. Coordinated activities of wild-type plus mutant EZH2 drive tumor-associated hypertrimethylation of lysine 27 on histone H3 (H3K27) in human B-cell lymphomas. Proc Natl Acad Sci U S A 2010;107:20980-5. [Crossref] [PubMed]
- Oricchio E, Katanayeva N, Donaldson MC, et al. Genetic and epigenetic inactivation of SESTRIN1 controls mTORC1 and response to EZH2 inhibition in follicular lymphoma. Sci Transl Med 2017;9:eaak9969 [Crossref] [PubMed]
- Zingg D, Arenas-Ramirez N, Sahin D, et al. The Histone Methyltransferase Ezh2 Controls Mechanisms of Adaptive Resistance to Tumor Immunotherapy. Cell Rep 2017;20:854-67. [Crossref] [PubMed]
- Mesin L, Ersching J, Victora GD. Germinal Center B Cell Dynamics. Immunity 2016;45:471-82. [Crossref] [PubMed]
- Morschhauser F, Tilly H, Chaidos A, et al. Tazemetostat for patients with relapsed or refractory follicular lymphoma: an open-label, single-arm, multicentre, phase 2 trial. Lancet Oncol 2020;21:1433-42. [Crossref] [PubMed]
- Ennishi D, Takata K, Béguelin W, et al. Molecular and Genetic Characterization of MHC Deficiency Identifies EZH2 as Therapeutic Target for Enhancing Immune Recognition. Cancer Discov 2019;9:546. [Crossref] [PubMed]
- Green MR, Kihira S, Liu CL, et al. Mutations in early follicular lymphoma progenitors are associated with suppressed antigen presentation. Proc Natl Acad Sci U S A 2015;112:E1116 [Crossref] [PubMed]
- Pasqualucci L, Dominguez-Sola D, Chiarenza A, et al. Inactivating mutations of acetyltransferase genes in B-cell lymphoma. Nature 2011;471:189-95. [Crossref] [PubMed]
- Zhang J, Vlasevska S, Wells VA, et al. The CREBBP Acetyltransferase Is a Haploinsufficient Tumor Suppressor in B-cell Lymphoma. Cancer Discov 2017;7:322. [Crossref] [PubMed]
- Hashwah H, Schmid CA, Kasser S, et al. Inactivation of CREBBP expands the germinal center B cell compartment, down-regulates MHCII expression and promotes DLBCL growth. Proc Natl Acad Sci U S A 2017;114:9701. [Crossref] [PubMed]
- Jiang Y, Ortega-Molina A, Geng H, et al. CREBBP Inactivation Promotes the Development of HDAC3-Dependent Lymphomas. Cancer Discov 2017;7:38-53. [Crossref] [PubMed]
- Mondello P, Tadros S, Teater M, et al. Selective inhibition of HDAC3 targets synthetic vulnerabilities and activates immune surveillance in lymphoma. Cancer Discov 2020;10:440-59. [Crossref] [PubMed]
- Riese RJ, Wolf PR, Brömme D, et al. Essential Role for Cathepsin S in MHC Class II–Associated Invariant Chain Processing and Peptide Loading. Immunity 1996;4:357-66. [Crossref] [PubMed]
- Shi G-P, Villadangos JA, Dranoff G, et al. Cathepsin S Required for Normal MHC Class II Peptide Loading and Germinal Center Development. Immunity 1999;10:197-206. [Crossref] [PubMed]
- Dheilly E, Battistello E, Katanayeva N, et al. Cathepsin S Regulates Antigen Processing and T Cell Activity in Non-Hodgkin Lymphoma. Cancer Cell 2020;37:674-89.e12. [Crossref] [PubMed]
- Bararia D, Hildebrand JA, Stolz S, et al. Cathepsin S Alterations Induce a Tumor-Promoting Immune Microenvironment in Follicular Lymphoma. Cell Rep 2020;31:107522 [Crossref] [PubMed]
- Pasero C, Speiser DE, Derré L, et al. The HVEM network: new directions in targeting novel costimulatory/co-inhibitory molecules for cancer therapy. Curr Opin Pharmacol 2012;12:478-85. [Crossref] [PubMed]
- Krieg C, Boyman O, Fu YX, et al. B and T lymphocyte attenuator regulates CD8+ T cell–intrinsic homeostasis and memory cell generation. Nat Immunol 2007;8:162-71. [Crossref] [PubMed]
- Launay E, Pangault C, Bertrand P, et al. High rate of TNFRSF14 gene alterations related to 1p36 region in de novo follicular lymphoma and impact on prognosis. Leukemia 2012;26:559-62. [Crossref] [PubMed]
- Cheung KJ, Johnson NA, Affleck JG, et al. Acquired TNFRSF14 mutations in follicular lymphoma are associated with worse prognosis. Cancer Res 2010;70:9166-74. [Crossref] [PubMed]
- Boice M, Salloum D, Mourcin F, et al. Loss of the HVEM Tumor Suppressor in Lymphoma and Restoration by Modified CAR-T Cells. Cell 2016;167:405-18.e13. [Crossref] [PubMed]
- Kotsiou E, Okosun J, Besley C, et al. TNFRSF14 aberrations in follicular lymphoma increase clinically significant allogeneic T-cell responses. Blood 2016;128:72-81. [Crossref] [PubMed]
- Carreras J, Lopez-Guillermo A, Kikuti YY, et al. High TNFRSF14 and low BTLA are associated with poor prognosis in Follicular Lymphoma and in Diffuse Large B-cell Lymphoma transformation. J Clin Exp Hematop 2019;59:1-16. [Crossref] [PubMed]
- A Phase 1b Open-label Study to Evaluate the Safety and Efficacy of CC-122 With Obinutuzumab (GA101) in Relapsed/Refractory DLBCL and iNHL. https://ClinicalTrials.gov/show/NCT02417285
- Pettitt AR, Barrington S, Kalakonda N, et al. NCRI petrea trial: a phase 3 evaluation of pet-guided, response-adapted therapy in patients with previously untreated, advanced-stage, high-tumour-burden follicular lymphoma. Hematol Oncol 2019;37:67-8. [Crossref]
- Trial of Hu5F9-G4 in Combination With Rituximab in Relapsed/Refractory B-cell Non-Hodgkin's Lymphoma. Available online: https://ClinicalTrials.gov/show/NCT02953509
- Advani R, Flinn I, Popplewell L, et al. CD47 Blockade by Hu5F9-G4 and Rituximab in Non-Hodgkin’s Lymphoma. N Engl J Med 2018;379:1711-21. [Crossref] [PubMed]
- Study of Lenalidomide, Venetoclax and Obinutuzumab in Patients With Treatment-Naïve Follicular Lymphoma. Available online:https://ClinicalTrials.gov/show/NCT03980171
-
. Available online: https://ClinicalTrials.gov/show/NCT02568553Lenalidomide and Blinatumomab for the Treatment of Relapsed Non-Hodgkin Lymphoma - A Study to Evaluate the Safety, Pharmacokinetics and Preliminary Anti-Tumor Activity of RO7227166 in Combination With Obinutuzumab and in Combination With Glofitamab Following a Pre-Treatment Dose of Obinutuzumab Administered in Participants With Relapsed/Refractory B-Cell Non-Hodgkin's Lymphoma. Available online: https://ClinicalTrials.gov/show/NCT04077723
- A Safety and Pharmacokinetic Study of BTCT4465A (Mosunetuzumab) as a Single Agent and Combined With Atezolizumab in Non-Hodgkin's Lymphoma (NHL) and Chronic Lymphocytic Leukemia (CLL). Available online: https://ClinicalTrials.gov/show/NCT02500407
-
. Available online: https://ClinicalTrials.gov/show/NCT03056339Umbilical & Cord Blood (CB) Derived CAR-Engineered NK Cells for B Lymphoid Malignancies - Liu E, Marin D, Banerjee P, et al. Use of CAR-Transduced Natural Killer Cells in CD19-Positive Lymphoid Tumors. N Engl J Med 2020;382:545-53. [Crossref] [PubMed]
- A Phase 2 Multicenter Study of Axicabtagene Ciloleucel in Subjects With Relapsed/Refractory Indolent Non-Hodgkin Lymphoma. Available online: https://ClinicalTrials.gov/show/NCT03105336
- Jacobson C, Chavez JC, Sehgal AR, et al. Primary Analysis of Zuma-5: A Phase 2 Study of Axicabtagene Ciloleucel (Axi-Cel) in Patients with Relapsed/Refractory (R/R) Indolent Non-Hodgkin Lymphoma (iNHL). Blood 2020;136:40-1.
- A Pilot Study of a Personalized Neoantigen Cancer Vaccine Following Front-Line Rituximab in Follicular Lymphoma. Available online: https://ClinicalTrials.gov/show/NCT03361852
- Oncoquest-L Vaccine in Patients With Previously Untreated Stage III or IV, Asymptomatic, Non-bulky Follicular Lymphoma. Available online: https://ClinicalTrials.gov/show/NCT02194751
-
. Available online: https://ClinicalTrials.gov/show/NCT03121677Personalized Tumor Vaccine Strategy and PD-1 Blockade in Patients With Follicular Lymphoma - Armand P, Zinzani PLL, Palcza J, et al. Phase 1-2 Study of Pembrolizumab Combined with the Anti-LAG-3 Antibody MK-4280 for the Treatment of Hematologic Malignancies. Blood 2019;134:1548. [Crossref]
-
. Available online: https://ClinicalTrials.gov/show/NCT03276468Evaluation of Atezolizumab-Venetoclax-Obinutuzumab Combination in Relapse/Refractory Lymphomas - Gribben JG, Fowler N, Morschhauser F. Mechanisms of Action of Lenalidomide in B-Cell Non-Hodgkin Lymphoma. J Clin Oncol 2015;33:2803-11. [Crossref] [PubMed]
- Ramsay AG, Clear AJ, Kelly G, et al. Follicular lymphoma cells induce T-cell immunologic synapse dysfunction that can be repaired with lenalidomide: implications for the tumor microenvironment and immunotherapy. Blood 2009;114:4713. [Crossref] [PubMed]
- McDaniel JM, Pinilla-Ibarz J, Epling-Burnette PK. Molecular action of lenalidomide in lymphocytes and hematologic malignancies. Adv Hematol 2012;2012:513702 [Crossref] [PubMed]
- Hayashi T, Hideshima T, Akiyama M, et al. Molecular mechanisms whereby immunomodulatory drugs activate natural killer cells: clinical application. Br J Haematol 2005;128:192-203. [Crossref] [PubMed]
- Vo DN, Alexia C, Allende-Vega N, et al. NK cell activation and recovery of NK cell subsets in lymphoma patients after obinutuzumab and lenalidomide treatment. OncoImmunology 2017;7:e1409322 [Crossref] [PubMed]
- Zhang L, Qian Z, Cai Z, et al. Synergistic antitumor effects of lenalidomide and rituximab on mantle cell lymphoma in vitro and in vivo. Am J Hematol 2009;84:553-9. [Crossref] [PubMed]
- Morschhauser F, Fowler NH, Feugier P, et al. Rituximab plus Lenalidomide in Advanced Untreated Follicular Lymphoma. N Engl J Med 2018;379:934-47. [Crossref] [PubMed]
- Leonard JP, Trneny M, Izutsu K, et al. AUGMENT: A Phase III Study of Lenalidomide Plus Rituximab Versus Placebo Plus Rituximab in Relapsed or Refractory Indolent Lymphoma. J Clin Oncol 2019;37:1188-99. [Crossref] [PubMed]
- Nastoupil LJ, Bijou F, Ribrag V, et al. Avadomide (CC-122), a Novel Cereblon Modulating Agent, Plus Rituximab in Patients with Relapsed or Refractory Follicular Lymphoma. Blood 2018;132:1602. [Crossref]
- Menter T, Tzankov A, Zucca E, et al. Prognostic implications of the microenvironment for follicular lymphoma under immunomodulation therapy. Br J Haematol 2020;189:707-17. [Crossref] [PubMed]
- Jaiswal S, Jamieson CHM, Pang WW, et al. CD47 Is Upregulated on Circulating Hematopoietic Stem Cells and Leukemia Cells to Avoid Phagocytosis. Cell 2009;138:271-85. [Crossref] [PubMed]
- Tseng D, Volkmer JP, Willingham SB, et al. Anti-CD47 antibody–mediated phagocytosis of cancer by macrophages primes an effective antitumor T-cell response. Proc Natl Acad Sci U S A 2013;110:11103. [Crossref] [PubMed]
- Nakamura K, Casey M, Oey H, et al. Targeting an adenosine-mediated "don't eat me signal" augments anti-lymphoma immunity by anti-CD20 monoclonal antibody. Leukemia 2020;34:2708-21. [Crossref] [PubMed]
- Green MR, Monti S, Rodig SJ, et al. Integrative analysis reveals selective 9p24. 1 amplification, increased PD-1 ligand expression, and further induction via JAK2 in nodular sclerosing Hodgkin lymphoma and primary mediastinal large B-cell lymphoma. Blood 2010;116:3268-77. [Crossref] [PubMed]
- Kataoka K, Shiraishi Y, Takeda Y, et al. Aberrant PD-L1 expression through 3'-UTR disruption in multiple cancers. Nature 2016;534:402-6. [Crossref] [PubMed]
- Ding W, Laplant B, Witzig TE, et al. PD-1 Blockade with Pembrolizumab in Relapsed Low Grade Non-Hodgkin Lymphoma. Blood 2017;130:4055.
- Nastoupil L, Westin JR, Fowler NH, et al. High Complete Response Rates with Pembrolizumab in Combination with Rituximab in Patients with Relapsed Follicular Lymphoma: Results of an Open-Label, Phase II Study. Blood 2017;130:414.
- Neelapu SS, Locke FL, Bartlett NL, et al. Axicabtagene Ciloleucel CAR T-Cell Therapy in Refractory Large B-Cell Lymphoma. N Engl J Med 2017;377:2531-44. [Crossref] [PubMed]
- Schuster SJ, Bishop MR, Tam CS, et al. Tisagenlecleucel in Adult Relapsed or Refractory Diffuse Large B-Cell Lymphoma. N Engl J Med 2019;380:45-56. [Crossref] [PubMed]
- Hirayama AV, Gauthier J, Hay KA, et al. High rate of durable complete remission in follicular lymphoma after CD19 CAR-T cell immunotherapy. Blood 2019;134:636-40. [Crossref] [PubMed]
- Curran KJ, Seinstra BA, Nikhamin Y, et al. Enhancing Antitumor Efficacy of Chimeric Antigen Receptor T Cells Through Constitutive CD40L Expression. Mol Ther 2015;23:769-78. [Crossref] [PubMed]
- Sun LL, Ellerman D, Mathieu M, et al. Anti-CD20/CD3 T cell–dependent bispecific antibody for the treatment of B cell malignancies. Sci Transl Med 2015;7:287ra70 [Crossref] [PubMed]
- Schuster SJ, Bartlett NL, Assouline S, et al. Mosunetuzumab Induces Complete Remissions in Poor Prognosis Non-Hodgkin Lymphoma Patients, Including Those Who Are Resistant to or Relapsing After Chimeric Antigen Receptor T-Cell (CAR-T) Therapies, and Is Active in Treatment through Multiple Lines. Blood 2019;134:6. [Crossref]
- Morschhauser F, Carlo-Stella C, Offner F, et al. Dual CD20-Targeted Therapy With Concurrent CD20-TCB and Obinutuzumab Shows Highly Promising Clinical Activity and Manageable Safety in Relapsed or Refractory B-Cell Non-Hodgkin Lymphoma: Preliminary Results From a Phase Ib Trial. Blood 2019;134:1584. [Crossref]
- Schumacher TN, Scheper W, Kvistborg P. Cancer Neoantigens. Annu Rev Immunol 2019;37:173-200. [Crossref] [PubMed]
- Strothmeyer A-M, Papaioannou D, Dühren-von Minden M, et al. Comparative analysis of predicted HLA binding of immunoglobulin idiotype sequences indicates T cell–mediated immunosurveillance in follicular lymphoma. Blood 2010;116:1734-6. [Crossref] [PubMed]
- Khodadoust MS, Olsson N, Chen B, et al. B-cell lymphomas present immunoglobulin neoantigens. Blood 2019;133:878-81. [Crossref] [PubMed]
- Nielsen JS, Sedgwick CG, Shahid A, et al. Toward Personalized Lymphoma Immunotherapy: Identification of Common Driver Mutations Recognized by Patient CD8+ T Cells. Clin Cancer Res 2016;22:2226-36. [Crossref] [PubMed]
- Mole RH. Whole body irradiation; radiobiology or medicine? Br J Radiol 1953;26:234-41. [Crossref] [PubMed]
- Ngwa W, Irabor OC, Schoenfeld JD, et al. Using immunotherapy to boost the abscopal effect. Nat Rev Cancer 2018;18:313-22. [Crossref] [PubMed]
- Barker HE, Paget JT, Khan AA, et al. The tumour microenvironment after radiotherapy: mechanisms of resistance and recurrence. Nat Rev Cancer 2015;15:409-25. [Crossref] [PubMed]
- MacManus MP, Hofman MS, Hicks RJ, et al. Abscopal Regressions of Lymphoma After Involved-Site Radiation Therapy Confirmed by Positron Emission Tomography. Int J Radiat Oncol Biol Phys 2020;108:204-11. [Crossref] [PubMed]
- Vatner RE, Cooper BT, Vanpouille-Box C, et al. Combinations of immunotherapy and radiation in cancer therapy. Front Oncol 2014;4:325. [Crossref] [PubMed]
- Hanahan D, Weinberg RA. Hallmarks of cancer: the next generation. Cell 2011;144:646-74. [Crossref] [PubMed]
Cite this article as: Nath K, Tsang H, Gandhi MK. Tumor microenvironment of follicular lymphoma. Ann Lymphoma 2021;5:28.