Primary central nervous system lymphoma: advances in MRI and PET imaging
Introduction
Primary central nervous system lymphoma (PCNSL) is a rare form of extra-nodal non-Hodgkin lymphoma (NHL) that accounts for 1–2% of all cases of NHL and 1–4% of all central nervous system (CNS) tumors (1,2). The incidence of PCNSL is higher in immunocompromised patients such as those with uncontrolled acquired immunodeficiency syndrome (3). The International PCNSL Collaborative Group (IPCG) defines disease involvement to be limited to the CNS, leptomeninges and the vitreoretinal space (4). Histopathology shows over 90% of PCNSL consists of perivascular aggregates of malignant diffuse large B-cells, with the remainder consisting of T-cell lymphomas, poorly characterized low-grade lymphomas, or Burkitt’s lymphomas (5). Although, histopathology is essential for confirmation of disease, noninvasive imaging assessment is important as it assists with tissue sampling, response assessment after therapy, staging, and plays a significant role in narrowing the differential diagnosis. Here we review established clinical imaging techniques and highlight emerging neuroimaging approaches that may further improve the management of PCNSL in immunocompetent patients.
Anatomical magnetic resonance imaging (MRI)
Gadolinium enhanced MRI remains the imaging modality of choice for the noninvasive assessment of CNS lesions. A majority (60–70%) of PCNSL presents as solitary supra-tentorial lesions involving the periventricular white matter. However, multifocal disease (30–40%) and involvement of the infra-tentorial compartment and spinal cord is not uncommon (6-8). Current IPCG response assessment recommendations rely heavily on contrast enhancement for baseline evaluation of therapeutic response. The size of enhancement, classically thought to represent the extent of tumor burden, is measured as the sum product diameter of T1 shortening on T1W-post gadolinium images (4,9). This is problematic in PCNSL as it is known to be a diffusely infiltrative disease, which can comprise components with both intact and disrupted blood brain barrier (BBB). As contrast enhancement serves as a sensitive test for detecting BBB disruption, this can under-represent true disease burden due to poor detection of the components with an intact BBB. Further, agents such as corticosteroids often alter the pattern of contrast enhancement, due to modulation of BBB permeability, when used as part of treatment regimen or used as a premedication (10). Similarly, histopathological correlation of imaging in autopsy studies have established that contrast enhancement underestimates the true tumor burden of PCNSL and supports the notion of PCNSL being a whole brain disease (11).
The clinical utility of MRI lies in its noninvasive versatility, accessibility, and reproducibility. Typically, most centers include anatomical based T1-weighted (T1W) (with and without contrast) and T2-weighted (T2W) images as well as physiological imaging sequences such as diffusion-weighted imaging (DWI), dynamic contrast enhanced (DCE), or dynamic susceptibility-weighted contrast (DSC) perfusion MR as part of the imaging repertoire.
T1W imaging
T1W contrast enhanced MRI (CE-MRI) is typically used to clinically define the presence and extent of disease for initial staging, and for response assessment after therapy is initiated. Immunocompetent patients with PCNSL typically present with avidly enhancing lesions that frequently cross the corpus callosum. The complete disappearance of all enhancing abnormalities following induction therapy has been termed a “complete” radiographic response, while “progressive” disease is defined radiographically by a 25% increase in enhancement when compared with baseline or best response, which corresponds with inferior outcomes (4). These over simplified two dimensional CE-MRI based criteria remain the test of choice for staging and response assessment for all PCNSL patients in clinical practice and in most clinical trials. In this context, a notable clinical “pitfall” is to not account for intrinsic T1 signal that may be mistaken for residual enhancement (12). IPCG criteria address this unique issue by defining a special type of response, namely complete response unconfirmed (CRu) (4). CRu includes patients who fulfill the criteria for CR but (i) are on steroid therapy or (ii) have a small but persistent enhancing abnormality on MRI related to biopsy or focal hemorrhage. If theses lesions do not evolve with time or when off therapy, they can be categorized as CR in retrospect. Similarly, steroid use may alter the pattern of enhancement and is thought to decrease diagnostic accuracy of biopsy specimens (12). However, the probability of non-diagnostic biopsies after a short course of pre-operative steroids may indeed be very low and this risk needs to be balanced against clinical findings. If pre-operative steroids are necessary, a stereotactic scan can confirm continued presence of an enhancing lesional target prior to tissue sampling (13).
Challenges with the use of T1W CE-MRI as a marker of clinical response assessment includes variations in sequence parameters, timing, and dosage of MRI contrast administration, which are well known to impact the degree of enhancement (14-16). As previously mentioned, it is now widely recognized that contrast enhancement is a metric of BBB dysfunction and not a direct representation of tumor burden. In addition, cases of entirely non-enhancing CNS lymphoma have been reported which may delay diagnosis and impeded response assessment (17).
Further, two dimensional measurements of response assessment can be challenging in cases with complex, multifocal lesions. In addition, factors such as differences in slice thickness and magnetic field strength need to be taken into account. This is particularly important when comparing to an historical data set as well in the setting of multicenter studies. In comparison to other CNS tumors, the inherent differences at initial presentation, natural history, and response to therapy, underscores the need for standardized MRI parameters for advancing the field of PCNSL (15,18).
T2W imaging
T2W images and fluid attenuated inversion recovery (FLAIR) sequences are routinely acquired during MRI examinations. Despite this, T2 signal abnormalities are not incorporated into the current IPCG response assessment criteria, and there is limited published data on the utility of this parameter in PCNSL. PCNSL frequently presents as iso or hypointense (compared to the cortex) lesions on T2W MRI images due to high cellularity that appears distinct from peritumoral edema (Figure 1) (19,20). Clinicians familiar with PCNSL are also aware of progressive white matter changes best seen on T2W MRIs, even when tumors continue to show response based on enhancement (17,21). The risk factors of developing these white matter changes include the elderly (age >60), those receiving neurotoxic chemotherapies, and those who have received prior radiation. Preclinical and clinical evidence suggest that impaired biopterin metabolism as well as accumulation of homocysteine and adenosine may be contributing to this phenomenon (22-24). Other authors have proposed that the impact of methotrexate on the glial cells may have an influence and have also suggested a correlation with neurocognitive outcomes (25).
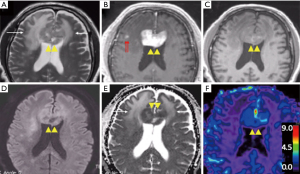
A recent retrospective report suggests over 60% of patients with high-dose methotrexate (HDMTX) based regimens may exhibit progressive T2 signal abnormalities (26). Interestingly, patients who received rituximab with methotrexate were more likely to have early and more extensive white matter changes compared to those that received HDMTX without rituximab. The clinical and prognostic implications of their findings are unclear but highlight the potential impact of therapeutic interventions on imaging.
Nonspecific T2 signal abnormalities are not uncommon, especially in the elderly and patients with comorbidities such as migraines. One retrospective study identified non-enhancing T2-FLAIR weighted hyperintense lesions at a distance from the enhancing lesion(s) in 23% of patients (27). Similarly, the significance of new non-enhancing T2 signal abnormalities for early diagnosis of disease relapse likely warrants further evaluation (28,29). The more recent consensus statement published on behalf of the IPCG recommends the acquisition of a contrast enhanced-T2W-FLAIR (CE-T2-FLAIR) sequence, in lieu of a contrast-enhanced T2W sequence, to be performed immediately following intravenous gadolinium contrast administration (30). The use of CE-T2W-FLAIR improves sensitivity for the detection of T1 and T2 hyperintense foci and may be most helpful in the evaluation of subtle lepto- and pachy-meningeal disease (30,31). Incorporation of advanced physiologic imaging may provide additional information in making the distinction between malignant and non-malignant T2 signal abnormalities.
Physiologic MRI
Diffusion-weighed imaging
Most centers with MRI capabilities include DWI in their standard protocol and allow the measurement of apparent diffusion coefficient (ADC) value. ADC is a sensitive measure of microscopic diffusion of unbound extracellular water molecules and is an extremely sensitive test when used to detect cerebral ischemia (32,33). ADC is also sensitive to detect the reduction of extracellular water diffusion due to densely packed proliferating tumors such as PCNSL (Figure 1D,E) (32-36). Therefore, ADC values can be clinically useful in the differentiation of PCNSL from other primary brain tumors (35). The potential clinical utility of this parameter has been tested in several retrospective studies that provide compelling evidence for an inverse correlation between ADC measurements and tumor cellular density, with higher ADC values being an excellent pre-treatment predictive biomarker for clinical outcomes such as progression-free survival (PFS) and overall survival (OS) after HDMTX based therapies (37-39). Similarly, patients with prolonged PFS and OS had a significant increase in post-therapeutic ADC values, and may reflect a true reduction in tumor burden compared to enhancing lesion size (39). In this context, other investigators, including a prospective phase II trial (n=52), did not find a significant difference in the predictive value of ADC (38-41). Like most primary brain tumors, intratumoral heterogeneity, susceptibility artifacts, and lack of anatomical clarity may dampen its predictive value in PCNSL. Nonetheless, DWI can provide useful information to aid in the neuroradiologic assessment of PCNSL.
Perfusion weighted imaging
MRI perfusion is an advanced technique that allows for quantitative assessment of tumor microvasculature. Two routinely utilized perfusion approaches are (I) T2* weighted DSC and (II) T1W DCE perfusion MRI. Both techniques utilize dynamic serial image acquisition over time before, during, and after contrast administration. DSC-MRI technique measures relative cerebral blood volume (rCBV) as a noninvasive imaging biomarker of tissue microvascular density. Meanwhile, DCE-MRI allows the measurement of the transfer constant (Ktrans), which is a mathematically derived value that reflects the leakage rate of contrast across the BBB (42,43).
DSC-MRI
Typically, more aggressive tumors (higher grade) have a higher degree of neoangiogenesis and are expected to have higher rCBV values (Figure 1F). However, retrospective evidence suggests that PCNSL patients (n=25) with low tumor rCBV values (<1.43) at pretherapy baseline are likely to have shorter PFS and OS compared with the patients with high tumor rCBV values (44). In the context of glioma, rCBV values have been widely studied and correlated with regards to histologic grade, prognosis, and clinical outcomes. In high grade gliomas, neoangiogenesis (e.g., microvascular proliferation) is incorporated as part of pathological grading system (5,45). As such, higher grade tumors typically exhibit microvascular proliferation, which frequently translates to higher rCBV values on DSC-MRI compared to other pathologies (46-50). However, the utility of DSC-MRI in PCNSL remains less clear (compared to glioma literature) (51). In PCNSL, Valles et al. suggest that the observed correlation between rCBV and outcome may be a reflection of a relative lack of tumor angiogenesis, which translates to fewer patent vessels that can deliver intravenous methotrexate to the tumor bed. The clinical utility of DSC-rCBV values may be further improved when combined with ADC values. Technical factors in the measurement of rCBV may also contribute to the observed correlations with clinical outcome. For instance, the moderate to high degree of BBB permeability in PCNSL, as evident by the avid contrast enhancement, may result in T1W leakage effects that underestimate rCBV values. Strategies such as the use of a preload dose (PLD) of gadolinium-based contrast, low flip angle, and mathematical leakage correction may mitigate these confounding effects (52-54). In this context, the use of alternate investigational MR contrast agents such as large molecular weight ultra-small superparamagnetic iron oxide (USPIO) nanoparticles may provide an alternative to measuring tumor microvasculature, without the need for leakage correction (55,56).
DCE-MRI
Among all DCE-MRI parameters, Ktrans has most consistently demonstrated its value in distinguishing differential diagnoses with higher Ktrans values noted in lymphoma when compared to other primary and metastatic tumors (57-60). A retrospective study (n=18) in immunocompetent PCNSL patients by Hatzoglou et al. showed that DCE-MRI parameters might also serve as predictive biomarkers (41). Here, the authors validated the work by Valles et al. by demonstrating that lower Ktrans values correlate with increased risk for rapid progression. Ktrans was prospectively evaluated in seven patients as part of a larger prospective phase II study evaluating a HDMTX based poly-chemotherapy regimen preceded by administration of NGR-human tumor necrosis factor (NGR-hTNF), a TNF-α derivative capable of targeting tumor blood vessels and increasing endothelial permeability (61). Investigators demonstrate an increased Ktrans value after a second dose, which correlated with cerebrospinal fluid (CSF) concentration of drug and an increased uptake of 99mTc-DTPA. This study suggests the utility of Ktrans as a noninvasive biomarker for tumor permeability in PCNSL. Another retrospective study (n=18) comparing ADC to DCE-MRI suggest that the enhancing component of primary CNS lymphoma was found to have significantly lower mean and relative ADC than the enhancing component of glioblastoma (GBM), but not significantly different relative 90th percentiles for Ktrans values (62). In this study, authors note that ADC was superior to DCE-MRI in differentiating primary CNS lymphoma from GBM. Other investigators have suggested the use of initial area under the curve (IAUC) derived from DCE-MRI which has better reproducibility when evaluated with ADC may better distinguish between PCNSL and atypical GBM (63).
Small sample sizes and inconsistent acquisition parameters along with relatively poor image resolution, susceptibility artifacts from bone, calcification, and blood products especially after biopsies and need for post processing may define ultimate clinical utility of perfusion imaging. Since perfusion imaging is already a standard sequence at most tertiary centers, investigators should strongly consider prospective collection and publishing these parameters when reporting prospective studies. Nonetheless, there remains limited data on DCE correlations with treatment response and outcome, and further studies are likely needed to help confirm the results of these previous studies.
Nuclear imaging and positron emission tomography (PET)
Physiologic differences between lymphoma and infectious lesions such as toxoplasmosis in the brain are a key aspect of pre-biopsy workup in CNS lymphoma patients with HIV. Noninvasive functional nuclear imaging modalities such as single-photon emission computed tomography (SPECT) and PET are being evaluated with mixed results (64-66). A meta-analysis of 26 manuscripts comparing SPECT to PET suggest that PET may have higher sensitivity and specificity than SPECT in this scenario. However, it is noted that studies using a quantitative approach tend to have higher sensitivity than those using a qualitative approach (66). Their role in the workup of immunocompetent patients is less clear.
Fluorodeoxyglucose (FDG)-PET
Radiolabeled glucose analog 18F-FDG is actively transported across the BBB and phosphorylated within cells; its uptake reflects the tissue glucose metabolism and is usually high in high-grade tumors and relatively low in low-grade tumors. PET imaging with the FDG has become the standard study for staging systemic NHL (67,68). There is increasing evidence to suggest that FDG-PET imaging may be more sensitive than a conventional CT of the chest, abdomen, and pelvis as well as bone marrow biopsies for detecting systemic disease in PCNSL (69). Recent prospective studies suggest a significantly higher FDG-uptake in CNS lymphomas (n=45) compared to other histologic diagnoses (n=23) (70). Similarly, other authors have suggested that metabolic PET imaging with agents such as FDG and 11C-methionin shows promise as a predictive biomarker and early detection of recurrences (71,72). This modality may be limited by availability in the community and cost, in addition to other factors such as timing of administration and concomitant medications (steroids, anesthetics etc.). The value of FDG-PET in the diagnosis and/or response assessment is investigational at this time, but offers significant value in early phase biomarker driven clinical trials.
Novel MR contrast agents
CE-MR provides a noninvasive tool for the assessment of BBB permeability. Gadolinium based MRI contrasts remain the clinical agent of choice for monitoring most CNS pathologies. More recently, there is an interest to develop new molecularly targeted contrast agents or agents capable of detecting pathological changes in the local tumor microenvironment. Ferumoxytol, a superparamagnetic iron-oxide nanoparticle (SPION), is an FDA approved medication for intravenous iron supplementation and has received orphan drug designation as an MRI contrast agent (56). In addition to being approved for use in patients with poor renal functions, due to its large molecular weight ferumoxytol is also used as a blood pool agent in the early phase (up to 24 hrs) after administration, where it can be used to develop high resolution rCBV maps using the steady state technique (73). Steady state imaging is not feasible with conventional gadolinium contrasts at delayed time points (over 24–48 h) after administration (56). Contrast enhancement is found on various clinically used T1W MRI sequences, which improves border delineation, allows for the assessment of lesion internal morphology, and may be helpful in diagnosing and monitoring PCNSL (55). Elegant preclinical and clinical work by the same group demonstrates that MR signal can be co-localized to areas with activated macrophage and astrocytes (74-76). In a small study, 26 patients with PCNSL who underwent ferumoxytol MRI went on to get tissue biopsy 24–72 hrs after administration. Fixed biopsy tissues were stained by immunohistochemical staining for the dextran coating on the SPION (Dx1 staining) and Perls’ stain. Hypointensity on delayed SPION T2W scans demonstrated that iron that is predominantly concentrated in macrophages and more diffused and variable in and around lymphoma cells suggesting that SPION imaging may be a useful biomarker to identify activated macrophages in PCNSL and may have important diagnostic, prognostic, and therapeutic implications (77). The relevance of this approach in PCNSL lies in the fact that unlike Gadolinium, ferumoxytol allows evaluation of the tumor microenvironment (vascularity and local innate immune response). Further evaluation in the context of emerging immunotherapy trials in PCNSL is needed.
Summary
Anatomical brain imaging remains a valuable tool that can be easily incorporated to existing diagnostic and response assessment criteria in PCNSL. However, Institutional imaging variation and inconsistent clinical trial reporting diminishes the reliability and reproducibility of most of these tools. In an attempt to standardize imaging practices and set biologically based recommendations for the use of MRI and PET imaging in the diagnosis and monitoring PCNSL, a guideline statement was recently published by the International Primary CNS Lymphoma Collaborative Group (IPCG) (30). This manuscript provides detailed imaging parameters that will facilitate the adoption of these recommendations in both research and clinical settings. To enhance clinical feasibility, authors have developed both “ideal” and “minimum standard” protocols that will facilitate widespread adoption. Table 1 summarizes the advantages and disadvantages of various imaging parameters discussed in this review.
Table 1
Imaging technique | Advantages | Disadvantages | References |
---|---|---|---|
T1W CE-MRI | ● Current standard of care | ● Sensitive metric for BBB dysfunction and not a direct representation of tumor burden | ( |
● High degree of versatility, accessibility, and reproducibility | ● Impacted by steroids | ||
● Easy adaptability into existing response assessment criteria in systemic oncology | |||
T2W images and FLAIR sequences | ● Easy accessibility | ● Nonspecific, difficult to distinguish progression from treatment effects | ( |
● Sensitive to changes in extracellular water content | |||
Diffusion-weighed MRI | ● Easy accessibility | ● Intra tumor heterogeneity | ( |
● Sensitive marker for packed proliferating tumors such as PCNSL | ● Susceptibile to artifacts of blood products | ||
● Lower ADC than GBM, metastasis, or demyelinating lesions | ● Low spatial resolution | ||
Perfusion MRI imaging | ● Quantitative assessment of tumor microvasculature and neoangiogeneis | ● Need for post processing such as leakage correction. lack of anatomical clarity | ( |
● Lower rCBVs than GBM and higher than demyelinating lesions | ● Susceptibile to artifacts of blood products | ||
● Low spatial resolution | |||
FDG PET scan | ● Measures tissue glucose metabolism | ● Limited by availability in the community and high cost | ( |
● Higher metabolism than GBM, hypometabolism in demyelinating lesions | ● Factors such as timing of administration and concomitant medications may impact results | ||
● Low signal to noise |
PCNSL, primary central nervous system lymphoma; T1W, T1-weighted; CE-MRI, contrast enhanced magnetic resonance imaging; T2W, T2-weighted; FLAIR, fluid attenuated inversion recovery; FDG, fluorodeoxyglucose; PET, positron emission tomography; ADC, apparent diffusion coefficient; GBM, glioblastoma; rCBV, relative cerebral blood volume; BBB, blood brain barrier.
Conclusions
CE-MRI remains the imaging modality of choice for diagnosis and monitoring patients with PCNSL. MR sequences such as DWI, perfusion, and other novel metabolic imaging techniques such as PET and SPECT are capable of providing additional biological information not capable with CE-MRI alone. These imaging biomarkers may better assess therapeutic response and provide prognostic information about clinical outcomes. High degree of reproducibility as well as better estimates of their sensitivity and specificity is essential for clinical implementation of advanced MRI parameters. However, these imaging metrics require further prospective evaluation and consideration in prospective clinical trial settings.
Acknowledgments
The authors would like to thank Ms. Sofia Gallamore for her administrative support.
Funding: Supported in part by grants 1K08CA237809-01A1 (RFB) and NS082609, CA221938, CA220378, Mayo Clinic Foundation (LSH).
Footnote
Provenance and Peer Review: This article was commissioned by the Guest Editors (Andrés J. M. Ferreri, Maurilio Ponzoni) for the series “Central Nervous System Lymphomas” published in Annals of Lymphoma. The article has undergone external peer review.
Conflicts of Interest: All authors have completed the ICMJE uniform disclosure form (available at https://dx.doi.org/10.21037/aol-20-53). The series “Central Nervous System Lymphomas” was commissioned by the editorial office without any funding or sponsorship. LSH reports that he is funded by NINDS: NS082609, NCI: CA221938, CA220378, CA250481, and that he received royalties and consulting fees from Imaging Biometrics, LLC, and he also served on medical advisory board for Imaging Biometrics. Dr. LSH holds the following patents: US Patent: 10909675 US Patent App.: 16/975,647; 16/764,837; 63/112,496; 62/684,096; 62/635,276; 62/588,096; 62/351,129; 62/239,642 International App.: PCT/US2019/019687; PCT/US2018/061887; 19757457.7; 18878374.0; Dr. LSH holds stock of Imaging Biometrics, LLC, and is a co-founder of Precision Oncology Insights. Dr. LSH declares that the above financial disclosures are not directly related to the material presented in the manuscript. NA reports that she received payments from Bayer Healthcare for educational support to Bayer courses, symposia, and that there is no direct conflict of interest relating to the manuscript. RFB Jr reports that he is funded by NIH NCI grant #1K08CA237809-01A1. The authors have no other conflicts of interest to declare.
Ethical Statement: The authors are accountable for all aspects of the work in ensuring that questions related to the accuracy or integrity of any part of the work are appropriately investigated and resolved.
Open Access Statement: This is an Open Access article distributed in accordance with the Creative Commons Attribution-NonCommercial-NoDerivs 4.0 International License (CC BY-NC-ND 4.0), which permits the non-commercial replication and distribution of the article with the strict proviso that no changes or edits are made and the original work is properly cited (including links to both the formal publication through the relevant DOI and the license). See: https://creativecommons.org/licenses/by-nc-nd/4.0/.
References
- Ostrom QT, Cioffi G, Gittleman H, et al. CBTRUS statistical report: primary brain and other central nervous system tumors diagnosed in the United States in 2012-2016. Neuro Oncol 2019;21:v1-100. [Crossref] [PubMed]
- Villano JL, Koshy M, Shaikh H, et al. Age, gender, and racial differences in incidence and survival in primary CNS lymphoma. Br J Cancer 2011;105:1414-8. [Crossref] [PubMed]
- Algazi AP, Kadoch C, Rubenstein JL. Biology and treatment of primary central nervous system lymphoma. Neurotherapeutics 2009;6:587-97. [Crossref] [PubMed]
- Abrey LE, Batchelor TT, Ferreri AJ, et al. Report of an international workshop to standardize baseline evaluation and response criteria for primary CNS lymphoma. J Clin Oncol 2005;23:5034-43. [Crossref] [PubMed]
- Sabattini E, Bacci F, Sagramoso C, et al. WHO classification of tumours of haematopoietic and lymphoid tissues in 2008: an overview. Pathologica 2010;102:83-7. [PubMed]
- Slone HW, Blake JJ, Shah R, et al. CT and MRI findings of intracranial lymphoma. AJR Am J Roentgenol 2005;184:1679-85. [Crossref] [PubMed]
- Jack CR Jr, Reese DF, Scheithauer BW. Radiographic findings in 32 cases of primary CNS lymphoma. AJR Am J Roentgenol 1986;146:271-6. [Crossref] [PubMed]
- Haldorsen IS, Espeland A, Larsson EM. Central nervous system lymphoma: characteristic findings on traditional and advanced imaging. AJNR Am J Neuroradiol 2011;32:984-92. [Crossref] [PubMed]
- Cheng G, Zhang J. Imaging features (CT, MRI, MRS, and PET/CT) of primary central nervous system lymphoma in immunocompetent patients. Neurol Sci 2019;40:535-42. [Crossref] [PubMed]
- Batchelor T, Loeffler JS. Primary CNS lymphoma. J Clin Oncol 2006;24:1281-8. [Crossref] [PubMed]
- Lai R, Rosenblum MK, DeAngelis LM. Primary CNS lymphoma: a whole-brain disease? Neurology 2002;59:1557-62. [Crossref] [PubMed]
- Porter AB, Giannini C, Kaufmann T, et al. Primary central nervous system lymphoma can be histologically diagnosed after previous corticosteroid use: a pilot study to determine whether corticosteroids prevent the diagnosis of primary central nervous system lymphoma. Ann Neurol 2008;63:662-7. [Crossref] [PubMed]
- Bullis CL, Maldonado-Perez A, Bowden SG, et al. Diagnostic impact of preoperative corticosteroids in primary central nervous system lymphoma. J Clin Neurosci 2020;72:287-91. [Crossref] [PubMed]
- Kaufmann TJ, Smits M, Boxerman J, et al. Consensus recommendations for a standardized brain tumor imaging protocol for clinical trials in brain metastases. Neuro Oncol 2020;22:757-72. [Crossref] [PubMed]
- Ellingson BM, Bendszus M, Boxerman J, et al. Consensus recommendations for a standardized Brain Tumor Imaging Protocol in clinical trials. Neuro Oncol 2015;17:1188-98. [PubMed]
- Furutani K, Harada M, Mawlan M, et al. Difference in enhancement between spin echo and 3-dimensional fast spoiled gradient recalled acquisition in steady state magnetic resonance imaging of brain metastasis at 3-T magnetic resonance imaging. J Comput Assist Tomogr 2008;32:313-9. [Crossref] [PubMed]
- Bowden SG, Munger DN, Thiessen J, et al. The clinical heterogeneity of entirely nonenhancing CNS lymphoma: a case series. CNS Oncol 2021;10:CNS67. [Crossref] [PubMed]
- Grommes C, Rubenstein JL, DeAngelis LM, et al. Comprehensive approach to diagnosis and treatment of newly diagnosed primary CNS lymphoma. Neuro Oncol 2019;21:296-305. [Crossref] [PubMed]
- Küker W, Nägele T, Korfel A, et al. Primary central nervous system lymphomas (PCNSL): MRI features at presentation in 100 patients. J Neurooncol 2005;72:169-77. [Crossref] [PubMed]
- Bühring U, Herrlinger U, Krings T, et al. MRI features of primary central nervous system lymphomas at presentation. Neurology 2001;57:393-6. [Crossref] [PubMed]
- Ayuso-Peralta L, Orti-Pareja M, Zurdo-Hernandez M, et al. Cerebral lymphoma presenting as a leukoencephalopathy. J Neurol Neurosurg Psychiatry 2001;71:243-6. [Crossref] [PubMed]
- Lai R, Abrey LE, Rosenblum MK, et al. Treatment-induced leukoencephalopathy in primary CNS lymphoma: a clinical and autopsy study. Neurology 2004;62:451-6. [Crossref] [PubMed]
- Millot F, Dhondt JL, Mazingue F, et al. Changes of cerebral biopterin and biogenic amine metabolism in leukemic children receiving 5 g/m2 intravenous methotrexate. Pediatr Res 1995;37:151-4. [Crossref] [PubMed]
- Wilkinson HA, Fujiwara T, Rosenfeld S. Synergistic effect between intraneoplastic methotrexate and radiation on experimental intracerebral rat gliosarcoma. Neurosurgery 1994;34:665-8; discussion 668. [PubMed]
- Gibson EM, Nagaraja S, Ocampo A, et al. Methotrexate chemotherapy induces persistent tri-glial dysregulation that underlies chemotherapy-related cognitive impairment. Cell 2019;176:43-55.e13. [Crossref] [PubMed]
- Estephan F, Ye X, Dzaye O, et al. White matter changes in primary central nervous system lymphoma patients treated with high-dose methotrexate with or without rituximab. J Neurooncol 2019;145:461-6. [Crossref] [PubMed]
- Tabouret E, Houillier C, Martin-Duverneuil N, et al. Patterns of response and relapse in primary CNS lymphomas after first-line chemotherapy: imaging analysis of the ANOCEF-GOELAMS prospective randomized trial. Neuro Oncol 2017;19:422-9. [PubMed]
- Fischer L, Koch A, Schlegel U, et al. Non-enhancing relapse of a primary CNS lymphoma with multiple diffusion-restricted lesions. J Neurooncol 2011;102:163-6. [Crossref] [PubMed]
- DeAngelis LM. Cerebral lymphoma presenting as a nonenhancing lesion on computed tomographic/magnetic resonance scan. Ann Neurol 1993;33:308-11. [Crossref] [PubMed]
- Barajas RF Jr, Politi LS, Anzalone N, et al. Consensus recommendations for MRI and PET imaging of primary central nervous system lymphoma: guideline statement from the International Primary CNS Lymphoma Collaborative Group (IPCG). Neuro Oncol 2021;23:1056-71. [Crossref] [PubMed]
- Fukuoka H, Hirai T, Okuda T, et al. Comparison of the added value of contrast-enhanced 3D fluid-attenuated inversion recovery and magnetization-prepared rapid acquisition of gradient echo sequences in relation to conventional postcontrast T1-weighted images for the evaluation of leptomeningeal diseases at 3T. AJNR Am J Neuroradiol 2010;31:868-73. [Crossref] [PubMed]
- Romano A, Bozzao A, Bonamini M, et al. Diffusion-weighted MR Imaging: clinical applications in neuroradiology. Radiol Med 2003;106:521-48. [PubMed]
- Le Bihan D, Turner R, Douek P, et al. Diffusion MR imaging: clinical applications. AJR Am J Roentgenol 1992;159:591-9. [Crossref] [PubMed]
- Schob S, Meyer J, Gawlitza M, et al. Diffusion-Weighted MRI Reflects Proliferative Activity in Primary CNS Lymphoma. PLoS One 2016;11:e0161386 [Crossref] [PubMed]
- Guo AC, Cummings TJ, Dash RC, et al. Lymphomas and high-grade astrocytomas: comparison of water diffusibility and histologic characteristics. Radiology. 2002;224:177-83. [Crossref] [PubMed]
- Mrugala MM, Rubenstein JL, et al. Insights into the biology of primary central nervous system lymphoma. Curr Oncol Rep 2009;11:73-80. [Crossref] [PubMed]
- Zhang Y, Zhang Q, Wang XX, et al. Value of pretherapeutic DWI in evaluating prognosis and therapeutic effect in immunocompetent patients with primary central nervous system lymphoma given high-dose methotrexate-based chemotherapy: ADC-based assessment. Clin Radiol 2016;71:1018-29. [Crossref] [PubMed]
- Huang WY, Wen JB, Wu G, et al. Diffusion-weighted imaging for predicting and monitoring primary central nervous system lymphoma treatment response. AJNR Am J Neuroradiol 2016;37:2010-8. [Crossref] [PubMed]
- Barajas RF Jr, Rubenstein JL, Chang JS, et al. Diffusion-weighted MR imaging derived apparent diffusion coefficient is predictive of clinical outcome in primary central nervous system lymphoma. AJNR Am J Neuroradiol 2010;31:60-6. [Crossref] [PubMed]
- Morris PG, Correa DD, Yahalom J, et al. Rituximab, methotrexate, procarbazine, and vincristine followed by consolidation reduced-dose whole-brain radiotherapy and cytarabine in newly diagnosed primary CNS lymphoma: final results and long-term outcome. J Clin Oncol 2013;31:3971-9. [Crossref] [PubMed]
- Hatzoglou V, Oh JH, Buck O, et al. Pretreatment dynamic contrast-enhanced MRI biomarkers correlate with progression-free survival in primary central nervous system lymphoma. J Neurooncol 2018;140:351-8. [Crossref] [PubMed]
- Petrella JR, Provenzale JM. MR perfusion imaging of the brain: techniques and applications. AJR Am J Roentgenol 2000;175:207-19. [Crossref] [PubMed]
- Essig M, Shiroishi MS, Nguyen TB, et al. Perfusion MRI: the five most frequently asked technical questions. AJR Am J Roentgenol 2013;200:24-34. [Crossref] [PubMed]
- Valles FE, Perez-Valles CL, Regalado S, et al. Combined diffusion and perfusion MR imaging as biomarkers of prognosis in immunocompetent patients with primary central nervous system lymphoma. AJNR Am J Neuroradiol 2013;34:35-40. [Crossref] [PubMed]
- Louis DN, Perry A, Reifenberger G, et al. The 2016 World Health Organization classification of tumors of the central nervous system: a summary. Acta Neuropathol 2016;131:803-20. [Crossref] [PubMed]
- Barajas RF Jr, Chang JS, Segal MR, et al. Differentiation of recurrent glioblastoma multiforme from radiation necrosis after external beam radiation therapy with dynamic susceptibility-weighted contrast-enhanced perfusion MR imaging. Radiology 2009;253:486-96. [Crossref] [PubMed]
- Hu LS, Baxter LC, Smith KA, et al. Relative cerebral blood volume values to differentiate high-grade glioma recurrence from posttreatment radiation effect: direct correlation between image-guided tissue histopathology and localized dynamic susceptibility-weighted contrast-enhanced perfusion MR imaging measurements. AJNR Am J Neuroradiol 2009;30:552-8. [Crossref] [PubMed]
- Aronen HJ, Gazit IE, Louis DN, et al. Cerebral blood volume maps of gliomas: comparison with tumor grade and histologic findings. Radiology 1994;191:41-51. [Crossref] [PubMed]
- Cha S, Lupo JM, Chen MH, et al. Differentiation of glioblastoma multiforme and single brain metastasis by peak height and percentage of signal intensity recovery derived from dynamic susceptibility-weighted contrast-enhanced perfusion MR imaging. AJNR Am J Neuroradiol 2007;28:1078-84. [Crossref] [PubMed]
- Barajas RF, Chang JS, Sneed PK, et al. Distinguishing recurrent intra-axial metastatic tumor from radiation necrosis following gamma knife radiosurgery using dynamic susceptibility-weighted contrast-enhanced perfusion MR imaging. AJNR Am J Neuroradiol 2009;30:367-72. [Crossref] [PubMed]
- Blasel S, Vorwerk R, Kiyose M, et al. New MR perfusion features in primary central nervous system lymphomas: pattern and prognostic impact. J Neurol. 2018;265:647-58. [Crossref] [PubMed]
- Boxerman JL, Schmainda KM, Weisskoff RM. Relative cerebral blood volume maps corrected for contrast agent extravasation significantly correlate with glioma tumor grade, whereas uncorrected maps do not. AJNR Am J Neuroradiol 2006;27:859-67. [PubMed]
- Boxerman JL, Prah DE, Paulson ES, et al. The Role of preload and leakage correction in gadolinium-based cerebral blood volume estimation determined by comparison with MION as a criterion standard. AJNR Am J Neuroradiol 2012;33:1081-7. [Crossref] [PubMed]
- Schmainda KM, Prah MA, Hu LS, et al. Moving toward a consensus DSC-MRI protocol: validation of a low-flip angle single-dose option as a reference standard for brain tumors. AJNR Am J Neuroradiol 2019;40:626-33. [Crossref] [PubMed]
- Farrell BT, Hamilton BE, Dosa E, et al. Using iron oxide nanoparticles to diagnose CNS inflammatory diseases and PCNSL. Neurology 2013;81:256-63. [Crossref] [PubMed]
- Toth GB, Varallyay CG, Horvath A, et al. Current and potential imaging applications of ferumoxytol for magnetic resonance imaging. Kidney Int 2017;92:47-66. [Crossref] [PubMed]
- Abe T, Mizobuchi Y, Nakajima K, et al. Diagnosis of brain tumors using dynamic contrast-enhanced perfusion imaging with a short acquisition time. Springerplus 2015;4:88. [Crossref] [PubMed]
- Murayama K, Nishiyama Y, Hirose Y, et al. Differentiating between central nervous system lymphoma and high-grade glioma using dynamic susceptibility contrast and dynamic contrast-enhanced MR imaging with histogram analysis. Magn Reson Med Sci 2018;17:42-9. [Crossref] [PubMed]
- Xi YB, Kang XW, Wang N, et al. Differentiation of primary central nervous system lymphoma from high-grade glioma and brain metastasis using arterial spin labeling and dynamic contrast-enhanced magnetic resonance imaging. Eur J Radiol 2019;112:59-64. [Crossref] [PubMed]
- Lu S, Gao Q, Yu J, et al. Utility of dynamic contrast-enhanced magnetic resonance imaging for differentiating glioblastoma, primary central nervous system lymphoma and brain metastatic tumor. Eur J Radiol 2016;85:1722-7. [Crossref] [PubMed]
- Ferreri AJM, Calimeri T, Ponzoni M, et al. Improving the antitumor activity of R-CHOP with NGR-hTNF in primary CNS lymphoma: final results of a phase 2 trial. Blood Adv 2020;4:3648-58. [Crossref] [PubMed]
- Lin X, Lee M, Buck O, et al. Diagnostic accuracy of T1-weighted dynamic contrast-enhanced-MRI and DWI-ADC for differentiation of glioblastoma and primary CNS lymphoma. AJNR Am J Neuroradiol 2017;38:485-91. [Crossref] [PubMed]
- Choi YS, Lee HJ, Ahn SS, et al. Primary central nervous system lymphoma and atypical glioblastoma: differentiation using the initial area under the curve derived from dynamic contrast-enhanced MR and the apparent diffusion coefficient. Eur Radiol 2017;27:1344-51. [Crossref] [PubMed]
- Miller RF, Hall-Craggs MA, et al. Magnetic resonance imaging, thallium-201 SPET scanning, and laboratory analyses for discrimination of cerebral lymphoma and toxoplasmosis in AIDS. Sex Transm Infect 1998;74:258-64. [Crossref] [PubMed]
- Antinori A, De Rossi G, Ammassari A, et al. Value of combined approach with thallium-201 single-photon emission computed tomography and Epstein-Barr virus DNA polymerase chain reaction in CSF for the diagnosis of AIDS-related primary CNS lymphoma. J Clin Oncol 1999;17:554-60. [Crossref] [PubMed]
- Yang M, Sun J, Bai HX, et al. Diagnostic accuracy of SPECT, PET, and MRS for primary central nervous system lymphoma in HIV patients: a systematic review and meta-analysis. Medicine (Baltimore) 2017;96:e6676 [Crossref] [PubMed]
- Cheson BD, Fisher RI, Barrington SF, et al. Recommendations for initial evaluation, staging, and response assessment of Hodgkin and non-Hodgkin lymphoma: the Lugano classification. J Clin Oncol 2014;32:3059-68. [Crossref] [PubMed]
- Barrington SF, Kluge R. FDG PET for therapy monitoring in Hodgkin and non-Hodgkin lymphomas. Eur J Nucl Med Mol Imaging 2017;44:97-110. [Crossref] [PubMed]
- Mohile NA, Deangelis LM, Abrey LE. The utility of body FDG PET in staging primary central nervous system lymphoma. Neuro Oncol 2008;10:223-8. [Crossref] [PubMed]
- Gupta M, Gupta T, Purandare N, et al. Utility of flouro-deoxy-glucose positron emission tomography/computed tomography in the diagnostic and staging evaluation of patients with primary CNS lymphoma. CNS Oncol 2019;8:CNS46. [Crossref] [PubMed]
- Ogawa T, Kanno I, Hatazawa J, et al. Methionine PET for follow-up of radiation therapy of primary lymphoma of the brain. Radiographics 1994;14:101-10. [Crossref] [PubMed]
- Palmedo H, Urbach H, Bender H, et al. FDG-PET in immunocompetent patients with primary central nervous system lymphoma: correlation with MRI and clinical follow-up. Eur J Nucl Med Mol Imaging 2006;33:164-8. [Crossref] [PubMed]
- Varallyay CG, Nesbit E, Fu R, et al. High-resolution steady-state cerebral blood volume maps in patients with central nervous system neoplasms using ferumoxytol, a superparamagnetic iron oxide nanoparticle. J Cereb Blood Flow Metab 2013;33:780-6. [Crossref] [PubMed]
- McConnell HL, Schwartz DL, Richardson BE, et al. Ferumoxytol nanoparticle uptake in brain during acute neuroinflammation is cell-specific. Nanomedicine 2016;12:1535-42. [Crossref] [PubMed]
- Horváth A, Varallyay CG, Schwartz D, et al. Quantitative comparison of delayed ferumoxytol T1 enhancement with immediate gadoteridol enhancement in high grade gliomas. Magn Reson Med 2018;80:224-30. [Crossref] [PubMed]
- Barajas RF, Hamilton BE, Schwartz D, et al. Combined iron oxide nanoparticle ferumoxytol and gadolinium contrast enhanced MRI define glioblastoma pseudoprogression. Neuro Oncol 2019;21:517-26. [Crossref] [PubMed]
- Ambady P, Netto JP, Woltjer R, et al. Pathological correlation of USPIO imaging in primary central nervous system lymphoma. Neurology 2016;86:P4.253.
Cite this article as: Ambady P, Hu LS, Politi LS, Anzalone N, Barajas RF Jr. Primary central nervous system lymphoma: advances in MRI and PET imaging. Ann Lymphoma 2021;5:27.