From pathobiology to targeted treatment in Epstein Barr virus related T cell and Natural Killer cell lymphoproliferative diseases
Introduction
Epstein Barr virus (EBV) is a ubiquitous herpes virus, which infects more than 95% of the world’s population (1). It was first discovered in the 1960s during investigations into an infectious agent responsible for endemic Burkitt’s lymphoma (2). It was later discovered that EBV was the causal agent for infectious mononucleosis (IM), although, for most of the world’s population, infection is acquired in early childhood and is usually asymptomatic. Transmission is through an oral-oral route, via infected saliva. EBV appears capable of bidirectional transmigration across the oral epithelium as well as direct infection of epithelial cells (3,4). EBV subsequently binds to B cells through CD21 leading to their infection, following which expression of the viral genome leads to proliferation and transformation. Passage of infected naïve B cells through the germinal centre leads to differentiation into a memory phenotype and concurrent downregulation of all viral gene expression to establish a lifelong persistence. The ability of EBV to trigger B cell transformation creates oncogenic potential and a subsequent association with several malignancies including diffuse large B cell lymphoma (DLBCL), Hodgkin’s lymphoma and post-transplant lymphoproliferative disorder (PTLD). However, EBV is also capable of infecting other tissues including T and NK cells where the same induction of proliferation can lead to lymphoproliferative disease (LPD) and subsequent lymphoma.
The mechanism by which EBV infects T cells is not fully understood as CD21 is not usually expressed on mature T cells. Coleman et al. demonstrated that EBV type 2 (EBV-2) was capable of infecting purified T cells leading to proliferation and cytokine expression (5). Subsequent work by the same group showed that CRISPR knockout or antibody blockade of CD21 could prevent EBV-2 infecting Jurkat T cells (6). However, the majority of the world’s population are infected with EBV type 1 (EBV-1) and this strain is responsible for all T/NK-LPDs (7,8). There are now various lines of evidence that suggest EBV infects CD21-expressing T precursors during thymic differentiation prior to the point of lineage commitment. Naïve T cells have been demonstrated to express CD21 on exit from the thymus (9). Southern blotting of EBV can show viral clonality in T and NK cells isolated from the same patient (8). Similarly, common somatic mutations can be identified in more than one lymphocyte lineage (8). All of which supports the concept that the cell of origin is an EBV infected lymphoid progenitor.
Common to all EBV related T/NK LPD is presentation with pronounced systemic inflammatory symptoms including associated haemophagocytic lymphohistiocytosis (HLH) which contributes to high morbidity and mortality. The speed of progression as well as lymphadenopathy, extra-nodal involvement and cutaneous features depends on the disease subtype. The revised 4th edition of the WHO classification of tumours of haematopoietic and lymphoid tissues recognises 8 separate diagnoses (10). This includes chronic active EBV (CAEBV), which has a systemic as well as two cutaneous forms, severe mosquito bite allergy (SMBA) and hydroa vacciniforme-like lymphoproliferative disorder (HVLPD), Systemic EBV positive T cell lymphoma, Aggressive NK leukaemia (ANKL), Extranodal NK/T cell lymphoma (ENKTL) and EBV+ HLH, which is recognised as an LPD in its own right. These disorders can be seen worldwide but are more common in Latin America and Asia and are rare in European populations (11).
Diagnostic criteria for EBV-associated LPDs have not always been clear, particularly because they are rare and it is difficult to identify the infected lineage, prove clonality or demonstrate the disease is not the result of severe viral infection using standard diagnostic techniques. Furthermore, the T/NK cell LPDs tend to be resistant to conventional pharmacological approaches further complicating management and leading to rapid disease progression. This review will focus on the pathophysiology of EBV related T/NK LPD and how this can lead to more targeted and efficacious therapeutic approaches.
The role of the EBV in T and NK cells
After primary B cell infection, EBV initiates the expression of a unique set of growth transforming genes including the virus-encoded nuclear antigens EBNA 1 (the virus genome maintenance protein), EBNAs 2, 3A, 3B, 3C, and –LP (transcriptional activators/regulators), the latent membrane proteins LMPs 1 and 2A/2B (signal transducers) and low levels of BHRF1 (a bcl2 homolog), plus the non-coding EBER RNAs (EBERs) and two blocks of microRNAs (the BHRF1- and BART-miRs). This growth transforming Latency III is highly immunogenic and vulnerable to immunosurveillance, therefore to establish lifelong persistence, the virus adopts a restricted Latency 0 expressing only the EBERs and BART-miRs. However, this reflects only two of several alternative forms of latency with increasingly restricted transcriptional programmes compared to Latency III (Table 1). Although first identified in EBV-positive lymphomas, Latency II and I reflect transcriptional programmes adopted by the virus during the establishment of Latency 0 in B cells. Latency III also typically occurs in immunosuppressed hosts, classically in the form of PTLD (12).
Table 1
Disease | Latency mode | Latent gene expression |
---|---|---|
B cell PTLD | Latency III | EBNA-1, -2, -3A, -3B, 3C, -LP, LMP1, LMP2a & b, BHRF1, EBER 1&2, BART miRNAs, BHRF1 miRNAs |
Hodgkin Lymphoma, diffuse large B cell lymphoma | Latency II | EBNA-1, LMP1, LMP2a&b, EBER1&2, BART miRNAs |
EBV T/NK LPD/lymphoma | Latency I/II | EBNA-1, LMP1 (variable), LMP2a & b (variable), LMP2b-TR1, EBER 1 & 2, BART miRNAs2, Abortive lytic replication3 |
Burkitt lymphoma | Latency I | EBNA-1, EBER 1 & 2, BART miRNAs |
Healthy carrier state | Latency 0 | EBER 1 & 2, BART miRNAs |
Latency I/II includes a number of latency profiles that are intermediates between Latency I and II with different degrees of LMP1 and/or LMP2a and/or LMP2b. 1, in some cases, LMP2b is expressed from the L2TR promoter in the terminal repeat region; 2, in some cases, deletions within the BART miRNA clusters 1 and 2; 3, Abortive lytic replication has been demonstrated ex vivo in EBV+ T/NK LPD. This has also been shown in other EBV associated B cell malignancies, although its role is not fully understood. EBV, Epstein Barr virus; LPD, lymphoproliferative disease; PTLD, post-transplant lymphoproliferative disorder.
EBV+ T/NK LPDs are generally characterised by a Latency II transcriptional programme; EBNA-1, LMP-1 and LMP-2b, EBERs and miRs, although at least half do not express LMP-1. However, a recent analysis of the EBV genome in infected cells from Japanese CAEBV patients revealed frequent point mutations and intragenic deletions across the genome. Interestingly, certain BART miRs that repress entry into the virus lytic cycle and several core replication genes including BALF5, BMRF1, BSLF1, BALF2 and BBLF4 were lost, yet the immediate early BZLF1 and BRLF1 remained intact, implicating a role for abortive lytic replication in the pathogenesis of EBV related malignancy (8). In keeping with this, early lytic gene expression including BILF1 and BZLF1 has been noted in otherwise latent tumours including in primary Burkitt lymphoma biopsies and in EBV+ T/NK cell lines (13,14). EBNA-1, LMP1 and LMP2 are never deleted, highlighting their essential role in viral genome maintenance and lymphomagenesis (15).
EBV miRs may also play a role in lymphomagenesis and immune evasion, although this has been better explored in B cell malignancies (16). The role of EBER RNA is not clear in T/NK LPDs, despite its universal high-level expression and lack of deletion or mutation in malignancy (16). This is despite EBER deficient viruses being capable of infecting and transforming B cells in experimental models. In CAEBV and EBV+ HLH, BART19-3p appears to be able to promote proliferation and suppress apoptosis via inhibition of the tumour suppressor adenomatous polyposis coli (APC) (17). BHRF1 and BART miRs are capable of downregulating the transporter associated with antigen processing (TAP), this impairs loading of MHC class I with viral antigens and therefore attenuates the CD8+ T cell response (18). Similarly, MHC class II ligand generation is impaired by miRs at multiple levels preventing CD4+ T cell recognition (19). Additionally, IL12 is downregulated by miRs therefore limiting Th1 differentiation (19). These pathways may play a crucial role in preventing immune surveillance despite strong cell mediated immunity in healthy EBV carriers, and may frustrate immunotherapy as will be discussed later (20).
While the exact mechanisms by which EBV latency I/II infection in T/NK cells induces development of LPD are not understood, EBV is capable of interacting with the host cell in a number of ways. LMP-1 can induce the NF-κB pathway, likely by signalling through the tumour necrosis factor (TNF) receptor (21,22). Genes expressed in the latent phase are capable of modulating cell death responses leading to apoptosis resistance (23). BARF1, through the MAPK/c-Jun signalling pathway and LMP-2A, by triggering activation of Ras/PI3K/Akt can induce expression of antiapoptotic bcl family proteins (24,25). EBV can also trigger epigenetic changes, by inducing hypermethylation and histone modification (26,27). While this has been better mechanistically described in the context of gastric and nasopharyngeal tumours, similar epigenetic changes are seen in EBV+ T/NK LPD (28).
Disease subtypes
Chronic active EBV
CAEBV is characterised by IM like symptoms lasting at least 3 months with EBV detectable in both the blood and tissue infiltrating lymphocytes (10). Cases are reported most frequently in Asia, where it can represent a third of EBV+ T/NK LPD cases, but are rare in the West. The phenotype also differs across ethnic groups, in Japan the disease is exclusively of T and NK cells (29), yet in the West B cell involvement is reported with equal frequency (30). CAEBV is usually a disease of childhood and adolescence and tends to arise after primary EBV infection. Clinical features and prognosis can vary significantly; some patients have stable indolent disease whereas others progress rapidly with organ failure and secondary lymphoma (31).
The cutaneous forms of CAEBV—SMBA and HVLPD present in childhood and can occur with or without systemic disease (11). In HVLPD presentation is with a blistering rash triggered by sun exposure, which on biopsy shows infiltration with EBV positive lymphocytes, most commonly with a γδ phenotype in limited disease or CD8+ cells in patients with systemic features (32). In SMBA the skin lesions are characterised by bullae at the site of a mosquito bite that then develop into necrotic lesions infiltrated with EBV+ NK cells (33). Cytokines such as INFγ and chemokines such as CXCL11 and CXCL9 released by skin infiltrating infected lymphocytes drive the pathology (34). Associated systemic CAEBV is more common in those with Central American or Asian ancestry and carries a worse prognosis than in Europeans who tend to present with limited disease (34,35). Mortality is associated with subsequent development of HLH or a more aggressive lymphoma and occurs 6–14 years after initial symptoms (33).
The drivers of CAEBV appear to relate to both impaired viral clearance combined with somatic and viral mutations driving abnormal lymphocyte proliferation. The INF-γ response towards EBV peptides is lower in cytotoxic T lymphocytes (CTLs) from CAEBV patients compared to healthy donors, patients with acute IM and healthy HLA-identical siblings (36,37). While there is an association with HLA-A26, this cannot explain the striking geographic variation and occurs despite CAEBV being, by definition, not associated with underlying immunodeficiency (11). The most frequent somatic mutations seen are in epigenetic regulators, particularly DDX3X, but also including KMT2D and TET2 (8). Similar changes are noted in more aggressive EBV T/NK LPD although CAEBV lacks other alterations such as JAK-STAT mutations. This mechanistically demonstrates how CAEBV can progress through the development of additional somatic mutations.
Treatment for CAEBV is generally unsatisfactory. Therapies such as immunosuppression, CTLs and rituximab for CD20+ disease tend to only cause transient improvement (38). The results are best achieved with allograft. In a cohort of 108 Japanese patients with CAEBV, 59 had an allograft of which 66% achieved long term cure (29). In the USA a smaller cohort 5/8 had long term cure post allograft whereas 3/8 developed progressive lymphoma (30). Timing of transplantation is a difficult issue; should it be done early prior to the development of severe end organ damage when outcomes are best, but with the knowledge that some patients have stable disease. The alternative approach is to watch and wait initially and proceed to transplant at the development of end organ damage, however, this risks higher complications from transplantation and an overall lesser chance of cure (38). Better understanding of which patients are likely to progress would help guide the use of allograft.
ANKL
ANKL is an extremely rare and rapidly progressive LPD which typically presents in the 3rd to 5th decade of life and is seen most frequently in Asia (10). Patients present with fever and cytopenias associated with a circulating clonal population of EBV infected NK cells (10). Presentation is frequently complicated with multiorgan failure and HLH which contributes to a median survival of less than two months (39). The immunophenotype is similar to ENKTL being CD2+, CD3−, CD5− and CD56+ although CD16 is more commonly positive (40,41). Diagnosis can be difficult as the EBV+ cells can be infrequent and a detailed phenotype can be difficult to assess with conventional immunohistochemistry and FISH, these difficulties are compounded by the rarity of the condition. FlowRNA, which allows detection of EBER+ cells by in situ hybridisation along with detailed phenotyping via flow cytometry, should make identifying cases less challenging (42). Otherwise, a diagnosis of ANKL rather than ENKTL, CAEBV or EBV+ HLH is made on a lack of nasal symptoms combined with circulating leukaemic cells and an aggressive clinical presentation.
Several studies have looked at somatic mutations in ANKL. Using whole exome sequencing recurrent mutations, which are usually activating, have been reported in the JAK-STAT and RAS-MAPK pathways (43). The exact genes involved are heterogenous, for example mutations are described in JAK2, STAT3 and STAT5, suggesting that dysregulation of the pathway is the primary issue (44). Mutations in epigenetic regulators are also common including ASXL1, TET2 and DNMT3A (45). In a large study combining genome sequencing with transcriptome analysis, dysregulation of the JAK-STAT-MYC axis was seen as the primary driver of ANKL; in around half of patients this was driven by direct mutations in pathway genes with an enrichment of epigenetic mutations such as TET2 in those with wild type JAK-STAT (46). It is also not clear what differentiates ANKL from less aggressive EBV T/NK LPD with a similar mutational burden although chromosome abnormalities such as 1q gain and 17p are reported more frequently in ANKL (46). The role of p53 mutations are also not clear and have been reported at variable frequencies by different groups (43,45).
In general, outcomes are dismal with no standard therapies or prospective data available to guide treatment. Asparaginase containing induction regimens, as used for ENKTL, are associated with improved outcomes compared to anthracycline based regimens in retrospective series (39). Only a small proportion of patients have a sufficient response to allow for allograft, the only curative option, even with this therapy survival is only 20–30% (47,48).
EBV related HLH
HLH is a multisystem hyperinflammatory disorder characterised by fever, cytopenias, hepatic dysfunction and organomegaly with multiple potential triggers (49). In adults, viral infections are the most frequent cause for HLH and EBV is the most common provoking virus, although EBV related HLH can occur at any age (50). EBV infected proliferating lymphocytes secrete cytokines such as IL-1b, IL-6, TNF-α IL-4, IFN-γ and IL-10 which drive hyperinflammation and activate bystander immune cells (51). Activated CD38+/HLA-DR+ T lymphocytes with type 1 polarisation appear to characterise reactive HLH, with this phenotype representing the virally infected cells when EBV is the driver (52).
The relationship between EBV and HLH can be complex and clinically difficult to determine; EBV can act as a trigger for primary HLH in children without representing an LPD and in adults EBV may be driving HLH indirectly through an underlying lymphoma which requires specific therapy (51). Conventional diagnostic tests, such as whole blood PCR, make it hard to determine the lineage or clonality of infected cells. This makes it difficult to identify an underlying cause, such as CAEBV, or a lineage marker, such as CD20, which would enable targeted treatment. Newer techniques such as flowRNA combining detailed phenotyping alongside detection of EBER should help solve some of these challenges (42).
EBV+ HLH is generally treated with dexamethasone and etoposide, according to the HLH-94 trial protocol (53), which aims to control the hyperinflammation through immunosuppression (54). Initial response rates in EBV related HLH are good, however, rapid relapse is a major issue with OS at five years around 25% (55). Immunosuppressive treatment may prevent an EBV specific immune response therefore creating an environment where EBV infected cells can proliferate unimpeded (42). Adding rituximab is a common practice despite the infected lineage usually being unknown, retrospective data has shown this reduces inflammation and viral load but there is no data showing improved overall survival (56,57). Allogenic stem cell transplant, which generates a graft v EBV+ lymphocyte effect, is the only curative option for relapsed EBV related HLH (49).
ENKTL
ENKTL is a cytotoxic T cell or NK cell malignancy occurring almost exclusively in association with EBV. It usually presents with necrotic and destructive extra-nodal lesions in the upper aerodigestive tract (10). In advanced disease spread is usually to other extra-nodal sites including the skin, GI tract and testis. Associated systemic symptoms such as fever and HLH are frequently observed. The diagnosis is more common in Asia and presents in the 4th and 5th decade of life (58).
Tumour cells are EBER positive with expression of latency II genes (10). The mutational burden is similar to ANKL with mutations in the JAK-STAT pathway as well as epigenetic regulators. Recurrent mutations have been identified in DDX3X, STAT3 and STAT5B, as well as MLL2, ARID1A, EP300 and ASXL3 (59). Compared to quiescent NK cells there is activation of the AKT, JAK/STAT and NFκB pathways. Dysregulation of these pathways happens at a greater frequency than gene mutations, potentially implicating EBV as a driver although the mechanism for this is not clear (60). Genes which are associated with interaction with the vascular endothelium are also overexpressed in ENKTL including VCAM1, CXCL9 and CXCL10 which may help to explain the degree of angioinvasion seen (60). Cytogenetically, 2q gain and 6q loss are frequently observed (46).
Historically outcomes for ENKTL have been poor with anthracycline based chemotherapy and a number of mechanisms have been explored to explain this (61). P-glycoprotein (P-gp), an efflux pump capable of excreting chemotherapeutic drugs, is frequently expressed in ENKTL (62). Knockdown of P-gp with siRNA can increase the sensitivity of cell lines to etoposide and doxorubicin. In a cohort of 105 ENKTL patients TP53 mutation was found in 14/105 via NGS and was associated with significantly worse prognosis (59). ENKTL cell lines also upregulate antiapoptotic proteins such as BCL-XL which can confer chemoresistance (13). Across a panel of ENKTL cell lines, despite variation in P-gp, as measured by rhodamine-123 efflux and p53 status, all were chemoresistant but responded to inhibition of bcl-2 family proteins (13).
Newer treatment regimens have improved outcomes of ENKTL and unlike other EBV related T/NK LPD there is prospective data to guide therapy. For localised disease the best outcomes are obtained with a combination of non-anthracycline based chemotherapy and radiotherapy with a 5-year OS of around 70% (63). The SMILE regimen (dexamethasone, methotrexate, ifosfamide, l-asparaginase, and etoposide) has greatly improved the success rates for treating advanced disease, with 5-year survival of 50% reported in a prospective trial (64). However, this regimen is associated with significant toxicity which can lead to dose delays and contributes to a treatment related mortality (TRM) rate of 6% (64). A newer regimen, DDGP (dexamethasone, cisplatin, gemcitabine, pegasparginase) was compared to SMILE in a RCT (n=42) leading to a 2-year OS of 74% versus 45% respectively, which could potentially be explained by better delivery of therapy to the DDGP group due to reduced toxicity (65).
Systemic EBV+ lymphoma of childhood
Systemic EBV positive lymphoma of childhood is an extremely rare disorder characterised by clonal expansion of EBV infected T cells (10). It can arise from primary EBV infection, when CD8+ disease predominates or CAEBV when the lineage is more commonly CD4+. This disorder is almost always associated with HLH and the progression to multiorgan failure and death is rapid (66). Historically the disease was referred to by a number of names, including sporadic fatal IM, before its nature as a lymphoproliferative disorder was known (10). It can be difficult to reach the diagnosis as the CD2+, CD3+, CD56–, TIA+ phenotype with clonal TCR rearrangement can be seen in EBV+ HLH and CAEBV. Biopsy of the bone marrow may only show subtle lymphoid infiltrates alongside more prominent haemophagocytosis (67). Organ biopsies can show a hepatic lymphoid infiltrate and depletion of the splenic white pulp, unfortunately, given the difficulty obtaining histology the diagnosis is often only reached at post-mortem (68). Given its rarity there are few studies looking at distinguishing genetic changes although it does appear that an abnormal karyotype is more common compared to EBV+ HLH and CAEBV (6).
Most patients are initially treated with a HLH based approach, which does not appear sufficient for disease control (67). SMILE chemotherapy, or other asparaginase based treatments, have been used prior to consolidation with an allogenic transplant (69). In general, even with transplantation, outcomes are poor with little data to base therapeutic decisions (67).
From pathology to therapy
Given the poor outcomes associated with EBV related T/NK LPD and their apparent intrinsic chemoresistance there is unmet need for new models of therapy. Potential approaches include reversing the lack of response to viral antigens with immunotherapy and targeting the pathway alterations and apoptosis resistance seen in virally infected cells (Figure 1). These approaches are counter to the immunosuppressive treatments traditionally used, which while capable of reducing hyperinflammation and improving organ function, can prevent immune surveillance against EBV leading to the subsequent risk of disease progression.
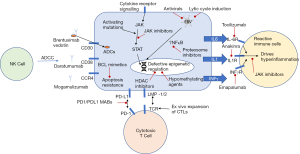
Checkpoint blockade
PD-1 checkpoint blockade has shown remarkable efficacy in treating multiple types of cancer, by overcoming T cell anergy and triggering an anti-tumour response (70). In the case of EBV+ T/NK LPD, it may provide a mechanism to reverse the lack of CTL response to the latency II antigens expressed by these tumours. PD-1L and PD-2L is expressed on tumour cells in around 60% of ENTLK cases with soluble PD-1L levels acting as a marker of disease stage and degree of viraemia (71). Structural variations (SV) in PD-L1, most commonly affecting the 3’ untranslated region (UTR), leading to elevated transcript levels, have been reported as a mechanism of immune escape in multiple cancers, although at a low level in more common subtypes of high-grade lymphoma (72). However, in EBV related lymphomas the frequency of PD-L1/L2 SVs is high, being present in 23% of ENKTL and 57% of ANKL cases and therefore representing one of the more commonly reported genetic variations (73). Although, there is also evidence that EBV can downregulate PD-L1 and PD-L2 by BHRF1-2-5p binding to the 3’-UTR of the PD-L1/L2 genes leading to reduced surface expression in lymphoblastoid cell lines (LCLs) (74). Given that overall EBV related lymphomas have increased PD-L1 expression this may represent a counterregulatory function, the relevance of this in T cell LPD is unknown. Overall, checkpoint expression is a potential mechanism by which a virally driven tumour can escape immune surveillance and is therefore a rational target for therapy.
A number of clinical trials have targeted the PD-1 axis. The largest, using the PD-1L blocking antibody avelumab in 21 patients with relapsed/refractory (r/r) ENKTL, showed encouraging results (75). While the overall response rate (ORR) was 38%, 21% achieved CR with a durable response. Smaller trials have shown similar results with anti-PD-1 antibodies in ENKTL and EBV+ HLH (76,77). Predictors of response include PD-1L expression and immune infiltration in the tumour microenvironment with responders showing an expansion of MAG3+ PD1+ CTLs capable of clearing the EBV+ clone (75). Other checkpoint inhibitors are in development, including those targeting CD47, TIM3 and LAG3, but as of yet there is no data in the context of EBV+ T/NK lymphomas (78,79).
While there is a rational concern in treating a condition characterised by inflammation with checkpoint blockade, crucially it is the malignant lymphocytes responsible for driving this process rather than the reacting immune system (42). Therefore, by eliminating the malignant cells the inflammatory picture should improve. This is borne out by the clinical data which has shown no concerning toxicity signals and resolution of HLH (75-77). There is also emerging evidence that PD-L1 expression on neutrophils worsens secondary inflammation in sepsis by promoting neutrophil survival, giving further theoretical reasons why targeting this axis could be beneficial (80). Checkpoint blockade also does not show overlapping toxicities with other agents in development, creating a rationale for combination therapy which may be able to increase the response rates reported in the trials described above.
Lymphoma targeting monoclonal antibodies (MABs)
Unlike in B cell lymphoma, which has been revolutionised with the anti-CD20 antibody rituximab, progress in the use of MABs in T cell lymphoma has been slower. The target which has generated the greatest interest in ENKTL is CD38. In myeloma, targeting CD38 with daratumumab has single agent efficacy and can improve responses when given in combination with other agents (81). This antigen is expressed in ENKTL, CAEBV and ANKL with strong expression being associated with worse outcomes (42,81). Initial case reports showed single agent efficacy in ENKTL, however, a phase II trial was disappointing with an ORR of only 25%, no CRs and a duration of response of only 55 days (82). A potential issue is that daratumumab relies on antibody dependent cellular cytotoxicity (ADCC) to exert its effect which may be inhibited by the immunosuppressive microenvironment which already prevents an immune response against EBV antigens. It is unclear if this drug may have a more significant role when combined with other agents such as checkpoint blockade.
CCR4 is another potential target for MAB therapy using mogamulizumab, which has shown efficacy in angioimmunoblastic T cell lymphoma (83). CCR4 is expressed on EBV infected T and NK cell lines as well as in primary samples, especially in the context of γδ LPD (84). Ex vivo mogamulizumab can induce ADCC against EBV+ cell lines and can slow tumour growth in an immunodeficient mouse model (84). However, there is no clinical data available and similar issues may exist to daratumumab with inducing ADCC in man.
Exploiting abnormal antigen expression to target cells with an antibody drug conjugate (ADC) is another potential therapeutic option which overcomes impaired ADCC. This strategy has been effective in other T cell lymphomas, particularly with brentuximab vedotin which uses a CD30 antibody to deliver the potent anti-tubule drug MMAE to malignant cells. A small trial looked at patients with relapsed/refractory EBV+ CD30+ lymphomas and found an ORR of 48% with a CR rate of 18%, which overall shows proof of concept (85). Designing better ADCs relies on combining an appropriate antigenic target with an effective payload and is of considerable interest (86).
Cytokine blockade
A wide variety of inflammatory cytokines are secreted by EBV infected T/NK cells, driving the resultant organ dysfunction. Suppressing this through targeted blockade is another potential therapeutic approach especially given the range of cytokine blocking drugs developed for other indications which could be repurposed for this role.
Tocilizumab, a MAB blocking the IL-6 receptor, was developed to treat rheumatoid arthritis but has shown efficacy in treating the cytokine release syndrome seen after chimeric antigen receptor T cell (CAR-T) therapy (87). It appears to be beneficial in improving markers of inflammation in critical illness induced HLH but has only been tested in small cohorts and not in the context of EBV (88). Anakinra, a recombinant IL-1 receptor antagonist, has also been used in the context of HLH in critically ill patients. In one study out of eight patients treated in the context of multiorgan failure, four survived to hospital discharge (89). Only a single patient had HLH provoked by EBV, but they rapidly died due to coagulopathy before any effect from treatment would be evident. Emapalumab, a monoclonal antibody against INF-λ, has an FDA licence for the treatment of relapsed/refractory primary HLH based on an ORR of 65% in a phase II/III study of 34 paediatric patients. There is no evidence in adults or in the context of secondary HLH, but this is currently being investigated in an actively recruiting clinical trial (NCT03985423).
Overall cytokine blockade seems to be a potential advantageous strategy, particularly given the poor organ function often seen at presentation with EBV T/NK LPD, to improve the patient’s performance status and allow more definitive therapy. However, this approach would need combining with other treatments capable of eliminating the malignant clone and there is a need for better clinical data to confirm efficacy.
Cellular immunotherapy
The success of allograft in treating EBV+ T/NK LPD demonstrates the potential benefit of cellular immunotherapy through a graft versus lymphoma effect (38,48). However, allograft comes at the context of significant toxicities and is most effective at consolidating rather than inducing remission (47). Using expanded antigen specific CTLs represents another mode of treatment, a number of groups have used LCLs to generate a polyclonal product targeting mainly EBNA-3A, 3B and 3C and early lytic antigens. With partial HLA matching it is possible to create a bank of EBV specific T cells from volunteer donors which can be available for rapid off the shelf use (90,91). This has proven effectiveness for the treatment of PTLD and a commercial product based on this technology, tabelecleucel, is completing late phase clinical testing NCT03392142. However, EBV+ T/NK LPDs develop in patients with intact immune systems with detectable immune responses to EBV antigens (42). In healthy EBV carriers CD8+ T cells are present with greater specificity towards lytic antigens, representing up to 2% of the cytotoxic T cell repertoire, then latent antigens (20). Among latent antigens a cytotoxic response to the EBNA 3s dominates. CD4+ T cells are present, but with a 10-fold lower response to the same antigen compared to CD8+ cells but are much less skewed towards lytic antigens. Although, it should be noted that patients with specific HLA-A genotypes possess much stronger responses towards antigens such as EBNA-2 (20). However, T/NK LPDs do not express the immune dominant EBNA-3s, instead expressing the subdominant LMP-1 and -2A/2TR and develop tumours despite intact immune function (92). Therefore, current off the shelf CTLs generated through LCLs may not have the same benefit as in PTLD. Although, this is the subject of ongoing clinical trials such as NCT04554914, testing tabelecleucel in a broad range of clinical indications including CAEBV.
There are however, a variety of methods to generate CTLs with better specificity towards latency II antigens in earlier stages of development, such as using LMP-1 peptides (93). Another approach is to transfect LCLs with LMP-1 and -2 and then use these cells for T cell expansion (94). A number of small trials tested CTLs manufactured this way, achieving modest response rates such as CR in 3/8 patients with R/R EBV+ T cell lymphoma. The same approach can be used to consolidate remission, using donor CTLs as post allograft consolidation leading to a 2-year OS of 60% in a single arm study (95). Donor CTLs were less successful in relapsed disease post allograft, which represents a very difficult to treat group, with only 2/5 patients briefly responding. Interestingly, a further 2/5 patients in this trial went on to respond to unselected donor lymphocyte infusion, potentially illustrating a need for broader antigen specificity than LMP. In keeping with this, in trials of banked CTLs in PTLD, there have been responses even when the tumour antigen does not correspond with TCR specificity of the product (96). This may relate to the presence of cells specific to other EBV antigens or stimulation of a non-specific immune response.
Given the success of CAR-T therapy against CD19 in B cell lymphoma there has been interest in developing a CAR against EBV antigens (97). This approach has been trialled in a nasopharyngeal cancer (NPC) setting using a second-generation CAR with an LMP-1 target (98). The transfected cells showed cytotoxicity against NPC cells in vitro and inhibited tumour growth in vivo in a mouse model. There is no published data in the lymphoma setting, however, there is much potential for development including using other EBV targets such as LMP-2 as well as alternative co-stimulatory domains to enhance the immune response.
One potential issue with cellular immunotherapy is again the immunosuppressive nature of the tumour microenvironment as well as a lack of antigen presentation. Myeloid derived suppressor cells (MDSCs) are expanded in EBV+ HLH and CAEBV (42). In the context of ENKTL MDSC expansion appears to be a negative prognostic marker (99). MDSCs from patients with EBV+ T/NK LPD can prevent T cell expansion. When combined with reduced antigen presentation via MHC class I and II due to EBV miRs and overexpression of PD-L1/L2 (16, 73); this may limit the success of cellular immunotherapy targeting viral antigens.
Targeting abnormal cellular pathways
EBV T/NK LPDs are characterised by abnormalities in the JAK/STAT and NF-κB pathways, defective epigenetic regulation and apoptosis resistance, all of which can be potentially targeted with small molecule inhibitors.
There has been interest in using bortezomib, a proteosome inhibitor, in ENKTL. NF-κB activation is inhibited by bortezomib via IκB phosphorylation, this is an important pathway of LMP-1 signalling in B cell disease although the role in EBV+ T/NK LPD is less clear (100). Bortezomib can induce apoptosis in EBV+ cell lines in vitro but clinical results have been poor. Combined with CHOP in a larger T cell lymphoma trial only 3/10 patients with ENKTL achieved CR with an association with early progression. It is not clear how much this was related to the CHOP backbone being insufficient for disease control. However, there was no correlation with expression of NF-κB and response, suggesting that this mechanism may not be clinically important (101).
HDAC inhibitors including romidepsin and belinostat have shown efficacy in peripheral T cell lymphoma (PTCL) (102). By altering gene expression HDAC inhibitors have multiple mechanisms of action including triggering apoptosis and growth arrest (102). A trial of romidepsin in ENKTL however had an unexpected result, 3/5 patients developed fever and liver impairment with a rising EBV titre (103). A similar rise in EBV DNA could be demonstrated in vitro from the supernatant of a SNK-6 cell line when treated with romdepsin which the authors proposed was due to induction of lytic replication. Some groups are interested in how this could allow for targeting of EBV with antiviral drugs, which will be discussed later.
Epigenetic changes in EBV T/NK malignancies are also seen via extensive promoter hypermethylation affecting multiple genes including TET2 and SOCS6 (45,104). Treatment of cell lines with the hypomethylating agent decitabine was associated with re-expression of silenced tumour suppressor genes. Hypomethylating agents have shown single agent efficacy in angioimmunoblastic T cell lymphoma (105) as well as safe combination with chemotherapy in PTCL giving evidence to support a trial in EBV+ T/NK LPD (106). Decitabine can also induce expression of latency III genes in the context of Burkitt lymphoma and therefore increase immunogenicity, although there is no data for a similar effect in T/NK LPD (107).
Targeting apoptotic pathways is another potential therapeutic approach, LMP1 signalling through NF-κB leads to upregulation of BCL-2, MCL-1 and BFL1/A1 conferring apoptosis resistance (23). BCL-2 inhibition has shown efficacy in the treatment of CLL and AML, however, venetoclax, a selective BCL-2 inhibitor is not effective in T and NK cell lines. However, targeting BCL-XL with a non-selective inhibitor, ABT-737 or the BCL-XL specific A-1331852 are highly effective in vitro and in a xenograft model (13). This effect is magnified by blocking BCL-XL in combination with MCL-1, although inhibiting multiple survival pathways may cause on target toxicity to healthy tissues, with myelotoxicity a significant issue. There is, as of yet, no clinical data studying BCL-XL inhibition alone or in combination in the context of EBV+ T/NK LPD.
The JAK/STAT pathway plays two roles in EBV+ T/NK LPD, firstly it is activated in the proliferating cells and appears to drive malignant expansion, and secondly it is the signalling pathway for the cytokines responsible for hyperinflammation and HLH. The licenced JAK 1/2 inhibitor ruxolitonib is effective in a mouse model of HLH by reducing STAT gene expression in response to cytokine stimulation without impacting lymphocyte cytotoxic function (108). There is only limited clinical data available using ruxolitonib as a single agent in HLH, indicating marked biochemical improvement without control of the underlying lymphoma, although this is not in the context of EBV+ T/NK LPD (109). A retrospective series examined combining ruxolitonib with dexamethasone, etoposide and liposomal doxorubicin (R-DED) in lymphoma associated HLH, of which approximately 60% of patients had ENKTL. Patients received this therapy for 1–2 weeks then went on to receive lymphoma subtype directed therapy without further ruxolitonib. Using resolution of HLH as an outcome, the ORR was 83.3% with R-DED compared to 54.8% in a historic HLH-94 treated control. Unfortunately, the cohort with ENKTL still did worse overall, while R-DED improved outcomes this was only an improvement in median OS from 1.5 to 5 months (110).
Targeting other members of the JAK family could potentially have greater anti-lymphoma effect. JAK3 is involved in signalling from the IL-2 receptor leading to STAT3 and STAT5 activation, with mutations and pathway alterations common in EBV+ T/NK LPD (111,112). The JAK 1/3 inhibitor tofacitinib inhibits STAT5 activation, leading to suppression of proliferation in EBV+ T/NK cell lines both in vitro and in xenografts. However, the drug does not fully suppress proliferation or stimulate significant apoptosis (113). PRN371, a highly selective JAK3 inhibitor can stimulate apoptosis, but cells lines with activating mutations in STAT3, such as SNK6, are resistant (114).
Antiviral strategies
Several groups have attempted to target EBV itself to treat the resultant LPD; this is problematic as standard antiviral drugs are not effective against latent virus. Nucleoside analogue antivirals require conversion to a monophosphate form by a viral thymidine kinase, expressed only in the lytic cycle (115). A potential strategy is therefore to trigger lytic replication alongside administering antivirals. Bortezomib, appears to be capable of inducing lytic replication in EBV infected B cells by inducing endoplasmic reticulum stress. A phase I trial attempted to exploit this by giving ganciclovir alongside bortezomib (NCT00093704), however, it appears only a single patient was recruited to this trial. As discussed HDAC inhibitors can also induce lytic cycle and this has been demonstrated the context of EBV+ B cell lymphoma and NPC where cell lines can be sensitised to the effect of ganciclovir, although HDAC inhibitors were toxic at micromolar concentrations as single agents (115).
A phase I/II trial of the pan-HDAC inhibitor arginine butyrate in combination with ganciclovir in the context of R/R EBV+ lymphoma, with 6/15 having T cell disease, showed a CR rate of 27%. The potential for newer more effective HDAC inhibitors to induce lytic cycle and show synergy with antivirals is not clear. It is also not clear how effective this will be in all patients given the frequency of mutations in the viral genome preventing true lytic cycle induction (15).
Conclusions
EBV+ T/NK LPDs are rare and aggressive disorders which respond poorly to conventional treatments. Greater understanding of the pathology, particularly with regard to apoptosis resistance, epigenetic changes and immune evasion have identified a number of therapeutic targets which are now in clinical trials or could be used in the near future. Response rates from single agents used so far, such as PD-1L blockade or JAK-STAT inhibition, however, remain low and it may be that effective combination therapy will be needed.
Acknowledgments
Funding: This work was supported by Cancer Research UK [C11497/A31309].
Footnote
Provenance and Peer Review: This article was commissioned by the editorial office, Annals of Lymphoma for the series “Lymphoma and Viruses”. The article has undergone external peer review
Conflicts of Interest: Both authors have completed the ICMJE uniform disclosure form (available at https://dx.doi.org/10.21037/aol-21-33). The series “Lymphoma and Viruses” was commissioned by the editorial office without any funding or sponsorship. CSL served as the unpaid guest editor of the series. The authors have no other conflicts of interest to declare.
Ethical Statement: The authors are accountable for all aspects of the work in ensuring that questions related to the accuracy or integrity of any part of the work are appropriately investigated and resolved.
Open Access Statement: This is an Open Access article distributed in accordance with the Creative Commons Attribution-NonCommercial-NoDerivs 4.0 International License (CC BY-NC-ND 4.0), which permits the non-commercial replication and distribution of the article with the strict proviso that no changes or edits are made and the original work is properly cited (including links to both the formal publication through the relevant DOI and the license). See: https://creativecommons.org/licenses/by-nc-nd/4.0/.
References
- Young LS, Yap LF, Murray PG. Epstein-Barr virus: more than 50 years old and still providing surprises. Nat Rev Cancer 2016;16:789-802. [Crossref] [PubMed]
- Epstein MA, Achong BG, Barr YM. Virus particles in cultured lymphoblasts from burkitt's lymphoma. Lancet 1964;1:702-3. [Crossref] [PubMed]
- Tugizov SM, Herrera R, Palefsky JM. Epstein-Barr virus transcytosis through polarized oral epithelial cells. J Virol 2013;87:8179-94. [Crossref] [PubMed]
- Tugizov SM, Berline JW, Palefsky JM. Epstein-Barr virus infection of polarized tongue and nasopharyngeal epithelial cells. Nat Med 2003;9:307-14. [Crossref] [PubMed]
- Coleman CB, Wohlford EM, Smith NA, et al. Epstein-Barr virus type 2 latently infects T cells, inducing an atypical activation characterized by expression of lymphotactic cytokines. J Virol 2015;89:2301-12. [Crossref] [PubMed]
- Smith NA, Coleman CB, Gewurz BE, et al. CD21 (Complement Receptor 2) Is the Receptor for Epstein-Barr Virus Entry into T Cells. J Virol 2020;94:e00428-20. [Crossref] [PubMed]
- Correa RM, Fellner MD, Alonio LV, et al. Epstein-barr virus (EBV) in healthy carriers: Distribution of genotypes and 30 bp deletion in latent membrane protein-1 (LMP-1) oncogene. J Med Virol 2004;73:583-8. [Crossref] [PubMed]
- Okuno Y, Murata T, Sato Y, et al. Defective Epstein-Barr virus in chronic active infection and haematological malignancy. Nat Microbiol 2019;4:404-13. [Crossref] [PubMed]
- Pekalski ML, García AR, Ferreira RC, et al. Neonatal and adult recent thymic emigrants produce IL-8 and express complement receptors CR1 and CR2. JCI Insight 2017;2:e93739. [Crossref] [PubMed]
- Swerdlow SH. WHO classification of tumours of haematopoietic and lymphoid tissues. Lyon: International Agency for Research on Cancer; 2008.
- Cohen JI, Iwatsuki K, Ko YH, et al. Epstein-Barr virus NK and T cell lymphoproliferative disease: report of a 2018 international meeting. Leuk Lymphoma 2020;61:808-19. [Crossref] [PubMed]
- Burns DM, Tierney R, Shannon-Lowe C, et al. Memory B-cell reconstitution following allogeneic hematopoietic stem cell transplantation is an EBV-associated transformation event. Blood 2015;126:2665-75. [Crossref] [PubMed]
- Sejic N, George LC, Tierney RJ, et al. BCL-XL inhibition by BH3-mimetic drugs induces apoptosis in models of Epstein-Barr virus-associated T/NK-cell lymphoma. Blood Adv 2020;4:4775-87. [PubMed]
- Tierney RJ, Shannon-Lowe CD, Fitzsimmons L, et al. Unexpected patterns of Epstein-Barr virus transcription revealed by a high throughput PCR array for absolute quantification of viral mRNA. Virology 2015;474:117-30. [Crossref] [PubMed]
- Murata T, Okuno Y, Sato Y, et al. Oncogenesis of CAEBV revealed: Intragenic deletions in the viral genome and leaky expression of lytic genes. Rev Med Virol 2020;30:e2095. [Crossref] [PubMed]
- Münz C. Immune Escape by Non-coding RNAs of the Epstein Barr Virus. Front Microbiol 2021;12:657387. [Crossref] [PubMed]
- Zhang Q, Luo D, Xie Z, et al. The Oncogenic Role of miR-BART19-3p in Epstein-Barr Virus-Associated Diseases. Biomed Res Int 2020;2020:5217039. [Crossref] [PubMed]
- Albanese M, Tagawa T, Bouvet M, et al. Epstein-Barr virus microRNAs reduce immune surveillance by virus-specific CD8+ T cells. Proc Natl Acad Sci U S A 2016;113:E6467-75. [Crossref] [PubMed]
- Tagawa T, Albanese M, Bouvet M, et al. Epstein-Barr viral miRNAs inhibit antiviral CD4+ T cell responses targeting IL-12 and peptide processing. J Exp Med 2016;213:2065-80. [Crossref] [PubMed]
- Long HM, Meckiff BJ, Taylor GS. The T-cell Response to Epstein-Barr Virus-New Tricks From an Old Dog. Front Immunol 2019;10:2193. [Crossref] [PubMed]
- Gires O, Zimber-Strobl U, Gonnella R, et al. Latent membrane protein 1 of Epstein-Barr virus mimics a constitutively active receptor molecule. EMBO J 1997;16:6131-40. [Crossref] [PubMed]
- Sun L, Zhao Y, Shi H, et al. LMP1 promotes nasal NK/T-cell lymphoma cell function by eIF4E via NF-κB pathway. Oncol Rep 2015;34:3264-71. [Crossref] [PubMed]
- Fitzsimmons L, Kelly GL. EBV and Apoptosis: The Viral Master Regulator of Cell Fate? Viruses 2017;9:339. [Crossref] [PubMed]
- Portis T, Longnecker R. Epstein-Barr virus (EBV) LMP2A mediates B-lymphocyte survival through constitutive activation of the Ras/PI3K/Akt pathway. Oncogene 2004;23:8619-28. [Crossref] [PubMed]
- Zhang Y, Xu M, Zhang X, et al. MAPK/c-Jun signaling pathway contributes to the upregulation of the anti-apoptotic proteins Bcl-2 and Bcl-xL induced by Epstein-Barr virus-encoded BARF1 in gastric carcinoma cells. Oncol Lett 2018;15:7537-44. [Crossref] [PubMed]
- Stanland LJ, Luftig MA. The Role of EBV-Induced Hypermethylation in Gastric Cancer Tumorigenesis. Viruses 2020;12:1222. [Crossref] [PubMed]
- Xie J, Wang Z, Fan W, et al. Targeting cancer cell plasticity by HDAC inhibition to reverse EBV-induced dedifferentiation in nasopharyngeal carcinoma. Signal Transduct Target Ther 2021;6:333. [Crossref] [PubMed]
- Küçük C, Wang J, Xiang Y, et al. Epigenetic aberrations in natural killer/T-cell lymphoma: diagnostic, prognostic and therapeutic implications. Ther Adv Med Oncol 2020;12:1758835919900856. [Crossref] [PubMed]
- Kimura H, Ito Y, Kawabe S, et al. EBV-associated T/NK-cell lymphoproliferative diseases in nonimmunocompromised hosts: prospective analysis of 108 cases. Blood 2012;119:673-86. [Crossref] [PubMed]
- Cohen JI, Jaffe ES, Dale JK, et al. Characterization and treatment of chronic active Epstein-Barr virus disease: a 28-year experience in the United States. Blood 2011;117:5835-49. [Crossref] [PubMed]
- Paik JH, Choe JY, Kim H, et al. Clinicopathological categorization of Epstein-Barr virus-positive T/NK-cell lymphoproliferative disease: an analysis of 42 cases with an emphasis on prognostic implications. Leuk Lymphoma 2017;58:53-63. [Crossref] [PubMed]
- Miyake T, Iwatsuki K, Hirai Y, et al. The aim of the measurement of Epstein-Barr virus DNA in hydroa vacciniforme and hypersensitivity to mosquito bites. J Med Virol 2020; [Epub ahead of print]. [Crossref] [PubMed]
- Tokura Y, Ishihara S, Tagawa S, et al. Hypersensitivity to mosquito bites as the primary clinical manifestation of a juvenile type of Epstein-Barr virus-associated natural killer cell leukemia/lymphoma. J Am Acad Dermatol 2001;45:569-78. [Crossref] [PubMed]
- Cohen JI, Manoli I, Dowdell K, et al. Hydroa vacciniforme-like lymphoproliferative disorder: an EBV disease with a low risk of systemic illness in whites. Blood 2019;133:2753-64. [Crossref] [PubMed]
- Quintanilla-Martinez L, Ridaura C, Nagl F, et al. Hydroa vacciniforme-like lymphoma: a chronic EBV+ lymphoproliferative disorder with risk to develop a systemic lymphoma. Blood 2013;122:3101-10. [Crossref] [PubMed]
- Xing Y, Song HM, Wei M, et al. Clinical significance of variations in levels of Epstein-Barr Virus (EBV) antigen and adaptive immune response during chronic active EBV infection in children. J Immunotoxicol 2013;10:387-92. [Crossref] [PubMed]
- Tsuge I, Morishima T, Kimura H, et al. Impaired cytotoxic T lymphocyte response to Epstein-Barr virus-infected NK cells in patients with severe chronic active EBV infection. J Med Virol 2001;64:141-8. [Crossref] [PubMed]
- Bollard CM, Cohen JI. How I treat T-cell chronic active Epstein-Barr virus disease. Blood 2018;131:2899-905. [Crossref] [PubMed]
- Ishida F, Ko YH, Kim WS, et al. Aggressive natural killer cell leukemia: therapeutic potential of L-asparaginase and allogeneic hematopoietic stem cell transplantation. Cancer Sci 2012;103:1079-83. [Crossref] [PubMed]
- Jiang NG, Jin YM, Niu Q, et al. Flow cytometric immunophenotyping is of great value to diagnosis of natural killer cell neoplasms involving bone marrow and peripheral blood. Ann Hematol 2013;92:89-96. [Crossref] [PubMed]
- Suzuki R, Suzumiya J, Nakamura S, et al. Aggressive natural killer-cell leukemia revisited: large granular lymphocyte leukemia of cytotoxic NK cells. Leukemia 2004;18:763-70. [Crossref] [PubMed]
- Collins PJ, Fox CP, George LC, et al. Characterising EBV-associated lymphoproliferative diseases and the role of myeloid-derived suppressor cells. Blood 2021;137:203-15. [Crossref] [PubMed]
- Dufva O, Kankainen M, Kelkka T, et al. Aggressive natural killer-cell leukemia mutational landscape and drug profiling highlight JAK-STAT signaling as therapeutic target. Nat Commun 2018;9:1567. [Crossref] [PubMed]
- El Hussein S, Patel KP, Fang H, et al. Genomic and Immunophenotypic Landscape of Aggressive NK-Cell Leukemia. Am J Surg Pathol 2020;44:1235-43. [Crossref] [PubMed]
- Huang L, Liu D, Wang N, et al. Integrated genomic analysis identifies deregulated JAK/STAT-MYC-biosynthesis axis in aggressive NK-cell leukemia. Cell Res 2018;28:172-86. [Crossref] [PubMed]
- Nakashima Y, Tagawa H, Suzuki R, et al. Genome-wide array-based comparative genomic hybridization of natural killer cell lymphoma/leukemia: different genomic alteration patterns of aggressive NK-cell leukemia and extranodal Nk/T-cell lymphoma, nasal type. Genes Chromosomes Cancer 2005;44:247-55. [Crossref] [PubMed]
- Hamadani M, Kanate AS, DiGilio A, et al. Allogeneic Hematopoietic Cell Transplantation for Aggressive NK Cell Leukemia. A Center for International Blood and Marrow Transplant Research Analysis. Biol Blood Marrow Transplant 2017;23:853-6. [Crossref] [PubMed]
- Fujimoto A, Ishida F, Izutsu K, et al. Allogeneic stem cell transplantation for patients with aggressive NK-cell leukemia. Bone Marrow Transplant 2021;56:347-56. [Crossref] [PubMed]
- La Rosée P, Horne A, Hines M, et al. Recommendations for the management of hemophagocytic lymphohistiocytosis in adults. Blood 2019;133:2465-77. [Crossref] [PubMed]
- Ramos-Casals M, Brito-Zerón P, López-Guillermo A, et al. Adult haemophagocytic syndrome. Lancet 2014;383:1503-16. [Crossref] [PubMed]
- Schram AM, Berliner N. How I treat hemophagocytic lymphohistiocytosis in the adult patient. Blood 2015;125:2908-14. [Crossref] [PubMed]
- Elazary AS, Wolf DG, Amir G, et al. Severe Epstein-Barr virus-associated hemophagocytic syndrome in six adult patients. J Clin Virol 2007;40:156-9. [Crossref] [PubMed]
- Henter JI, Samuelsson-Horne A, Aricò M, et al. Treatment of hemophagocytic lymphohistiocytosis with HLH-94 immunochemotherapy and bone marrow transplantation. Blood 2002;100:2367-73. [Crossref] [PubMed]
- Bergsten E, Horne A, Aricó M, et al. Confirmed efficacy of etoposide and dexamethasone in HLH treatment: long-term results of the cooperative HLH-2004 study. Blood 2017;130:2728-38. [Crossref] [PubMed]
- Beutel K, Gross-Wieltsch U, Wiesel T, et al. Infection of T lymphocytes in Epstein-Barr virus-associated hemophagocytic lymphohistiocytosis in children of non-Asian origin. Pediatr Blood Cancer 2009;53:184-90. [Crossref] [PubMed]
- Ioannou P, Akoumianaki E, Alexakis K, et al. Hemophagocytic lymphohistiocytosis syndrome associated with Epstein-Barr infection in an immunocompetent patient. A case study. Germs 2020;10:266-71. [Crossref] [PubMed]
- Birndt S, Schenk T, Heinevetter B, et al. Hemophagocytic lymphohistiocytosis in adults: collaborative analysis of 137 cases of a nationwide German registry. J Cancer Res Clin Oncol 2020;146:1065-77. [Crossref] [PubMed]
- Jeong SH. Extranodal NK/T cell lymphoma. Blood Res 2020;55:S63-71. [Crossref] [PubMed]
- Jiang L, Gu ZH, Yan ZX, et al. Exome sequencing identifies somatic mutations of DDX3X in natural killer/T-cell lymphoma. Nat Genet 2015;47:1061-6. [Crossref] [PubMed]
- Huang Y, de Reyniès A, de Leval L, et al. Gene expression profiling identifies emerging oncogenic pathways operating in extranodal NK/T-cell lymphoma, nasal type. Blood 2010;115:1226-37. [Crossref] [PubMed]
- Kim WS, Song SY, Ahn YC, et al. CHOP followed by involved field radiation: is it optimal for localized nasal natural killer/T-cell lymphoma? Ann Oncol 2001;12:349-52. [Crossref] [PubMed]
- Yoshimori M, Takada H, Imadome K, et al. P-glycoprotein is expressed and causes resistance to chemotherapy in EBV-positive T-cell lymphoproliferative diseases. Cancer Med 2015;4:1494-504. [Crossref] [PubMed]
- Yamaguchi M, Tobinai K, Oguchi M, et al. Phase I/II study of concurrent chemoradiotherapy for localized nasal natural killer/T-cell lymphoma: Japan Clinical Oncology Group Study JCOG0211. J Clin Oncol 2009;27:5594-600. [Crossref] [PubMed]
- Yamaguchi M, Kwong YL, Kim WS, et al. Phase II study of SMILE chemotherapy for newly diagnosed stage IV, relapsed, or refractory extranodal natural killer (NK)/T-cell lymphoma, nasal type: the NK-Cell Tumor Study Group study. J Clin Oncol 2011;29:4410-6. [Crossref] [PubMed]
- Li X, Cui Y, Sun Z, et al. DDGP versus SMILE in Newly Diagnosed Advanced Natural Killer/T-Cell Lymphoma: A Randomized Controlled, Multicenter, Open-label Study in China. Clin Cancer Res 2016;22:5223-8. [Crossref] [PubMed]
- Chen G, Chen L, Qin X, et al. Systemic Epstein-Barr virus positive T-cell lymphoproliferative disease of childhood with hemophagocytic syndrome. Int J Clin Exp Pathol 2014;7:7110-3. [PubMed]
- Coffey AM, Lewis A, Marcogliese AN, et al. A clinicopathologic study of the spectrum of systemic forms of EBV-associated T-cell lymphoproliferative disorders of childhood: A single tertiary care pediatric institution experience in North America. Pediatr Blood Cancer 2019;66:e27798. [Crossref] [PubMed]
- Keow JY, Stecho WM, Haig AR, et al. EBV-positive T/NK-associated lymphoproliferative disorders of childhood: A complete autopsy report. Indian J Pathol Microbiol 2020;63:78-82. [Crossref] [PubMed]
- Yoshida M, Osumi T, Imadome KI, et al. Successful treatment of systemic EBV positive T-cell lymphoma of childhood using the SMILE regimen. Pediatr Hematol Oncol 2018;35:121-4. [Crossref] [PubMed]
- Cristescu R, Mogg R, Ayers M, et al. Pan-tumor genomic biomarkers for PD-1 checkpoint blockade-based immunotherapy. Science 2018;362:eaar3593. [Crossref] [PubMed]
- Feng Y, Jing C, Yu X, et al. Predicting treatment response of patients with extranodal natural killer/T-cell lymphoma based on levels of PD-L1 mRNA and soluble PD-L1. Hematol Oncol 2020;38:467-77. [Crossref] [PubMed]
- Kataoka K, Shiraishi Y, Takeda Y, et al. Aberrant PD-L1 expression through 3'-UTR disruption in multiple cancers. Nature 2016;534:402-6. [Crossref] [PubMed]
- Kataoka K, Miyoshi H, Sakata S, et al. Frequent structural variations involving programmed death ligands in Epstein-Barr virus-associated lymphomas. Leukemia 2019;33:1687-99. [Crossref] [PubMed]
- Cristino AS, Nourse J, West RA, et al. EBV microRNA-BHRF1-2-5p targets the 3'UTR of immune checkpoint ligands PD-L1 and PD-L2. Blood 2019;134:2261-70. [Crossref] [PubMed]
- Kim SJ, Lim JQ, Laurensia Y, et al. Avelumab for the treatment of relapsed or refractory extranodal NK/T-cell lymphoma: an open-label phase 2 study. Blood 2020;136:2754-63. [Crossref] [PubMed]
- Kwong YL, Chan TSY, Tan D, et al. PD1 blockade with pembrolizumab is highly effective in relapsed or refractory NK/T-cell lymphoma failing l-asparaginase. Blood 2017;129:2437-42. [Crossref] [PubMed]
- Liu P, Pan X, Chen C, et al. Nivolumab treatment of relapsed/refractory Epstein-Barr virus-associated hemophagocytic lymphohistiocytosis in adults. Blood 2020;135:826-33. [Crossref] [PubMed]
- Jain S, Van Scoyk A, Morgan EA, et al. Targeted inhibition of CD47-SIRPalpha requires Fc-FcgammaR interactions to maximize activity in T-cell lymphomas. Blood. 2019;134:1430-40. [Crossref] [PubMed]
- Chen BJ, Dashnamoorthy R, Galera P, et al. The immune checkpoint molecules PD-1, PD-L1, TIM-3 and LAG-3 in diffuse large B-cell lymphoma. Oncotarget 2019;10:2030-40. [Crossref] [PubMed]
- Wang JF, Wang YP, Xie J, et al. Upregulated PD-L1 delays human neutrophil apoptosis and promotes lung injury in an experimental animal model of sepsis. Blood. 2021;
- Wang L, Wang H, Li PF, et al. CD38 expression predicts poor prognosis and might be a potential therapy target in extranodal NK/T cell lymphoma, nasal type. Ann Hematol 2015;94:1381-8. [Crossref] [PubMed]
- Morandi F, Horenstein AL, Costa F, et al. CD38: A Target for Immunotherapeutic Approaches in Multiple Myeloma. Front Immunol 2018;9:2722. [Crossref] [PubMed]
- Makita S, Tobinai K. Mogamulizumab for the treatment of T-cell lymphoma. Expert Opin Biol Ther 2017;17:1145-53. [Crossref] [PubMed]
- Kanazawa T, Hiramatsu Y, Iwata S, et al. Anti-CCR4 monoclonal antibody mogamulizumab for the treatment of EBV-associated T- and NK-cell lymphoproliferative diseases. Clin Cancer Res 2014;20:5075-84. [Crossref] [PubMed]
- Kim M, Lee JO, Koh J, et al. A phase II study of brentuximab vedotin in patients with relapsed or refractory Epstein-Barr virus-positive and CD30-positive lymphomas. Haematologica 2021;106:2277-80. [Crossref] [PubMed]
- Chu Y, Zhou X, Wang X. Antibody-drug conjugates for the treatment of lymphoma: clinical advances and latest progress. J Hematol Oncol 2021;14:88. [Crossref] [PubMed]
- Kotch C, Barrett D, Teachey DT. Tocilizumab for the treatment of chimeric antigen receptor T cell-induced cytokine release syndrome. Expert Rev Clin Immunol 2019;15:813-22. [Crossref] [PubMed]
- Dufranc E, Del Bello A, Belliere J, et al. IL6-R blocking with tocilizumab in critically ill patients with hemophagocytic syndrome. Crit Care 2020;24:166. [Crossref] [PubMed]
- Wohlfarth P, Agis H, Gualdoni GA, et al. Interleukin 1 Receptor Antagonist Anakinra, Intravenous Immunoglobulin, and Corticosteroids in the Management of Critically Ill Adult Patients With Hemophagocytic Lymphohistiocytosis. J Intensive Care Med 2019;34:723-31. [Crossref] [PubMed]
- Haque T, Wilkie GM, Jones MM, et al. Allogeneic cytotoxic T-cell therapy for EBV-positive posttransplantation lymphoproliferative disease: results of a phase 2 multicenter clinical trial. Blood 2007;110:1123-31. [Crossref] [PubMed]
- Prockop S, Doubrovina E, Suser S, et al. Off-the-shelf EBV-specific T cell immunotherapy for rituximab-refractory EBV-associated lymphoma following transplantation. J Clin Invest 2020;130:733-47. [Crossref] [PubMed]
- Fox CP, Haigh TA, Taylor GS, et al. A novel latent membrane 2 transcript expressed in Epstein-Barr virus-positive NK- and T-cell lymphoproliferative disease encodes a target for cellular immunotherapy. Blood 2010;116:3695-704. [Crossref] [PubMed]
- Hong J, Ni J, Ruan M, et al. LMP1-specific cytotoxic T cells for the treatment of EBV-related post-transplantation lymphoproliferative disorders. Int J Hematol 2020;111:851-7. [Crossref] [PubMed]
- Bollard CM, Gottschalk S, Torrano V, et al. Sustained complete responses in patients with lymphoma receiving autologous cytotoxic T lymphocytes targeting Epstein-Barr virus latent membrane proteins. J Clin Oncol 2014;32:798-808. [Crossref] [PubMed]
- McLaughlin LP, Rouce R, Gottschalk S, et al. EBV/LMP-specific T cells maintain remissions of T- and B-cell EBV lymphomas after allogeneic bone marrow transplantation. Blood 2018;132:2351-61. [Crossref] [PubMed]
- McAulay KA, Haque T, Urquhart G, et al. Epitope specificity and clonality of EBV-specific CTLs used to treat posttransplant lymphoproliferative disease. J Immunol 2009;182:3892-901. [Crossref] [PubMed]
- Neelapu SS, Locke FL, Bartlett NL, et al. Axicabtagene Ciloleucel CAR T-Cell Therapy in Refractory Large B-Cell Lymphoma. N Engl J Med 2017;377:2531-44. [Crossref] [PubMed]
- Tang X, Zhou Y, Li W, et al. T cells expressing a LMP1-specific chimeric antigen receptor mediate antitumor effects against LMP1-positive nasopharyngeal carcinoma cells in vitro and in vivo. J Biomed Res 2014;28:468-75. [PubMed]
- Zhang H, Li ZL, Ye SB, et al. Myeloid-derived suppressor cells inhibit T cell proliferation in human extranodal NK/T cell lymphoma: a novel prognostic indicator. Cancer Immunol Immunother 2015;64:1587-99. [Crossref] [PubMed]
- Iwata S, Yano S, Ito Y, et al. Bortezomib induces apoptosis in T lymphoma cells and natural killer lymphoma cells independent of Epstein-Barr virus infection. Int J Cancer 2011;129:2263-73. [Crossref] [PubMed]
- Kim SJ, Yoon DH, Kang HJ, et al. Bortezomib in combination with CHOP as first-line treatment for patients with stage III/IV peripheral T-cell lymphomas: a multicentre, single-arm, phase 2 trial. Eur J Cancer 2012;48:3223-31. [Crossref] [PubMed]
- Lopez AT, Bates S, Geskin L. Current Status of HDAC Inhibitors in Cutaneous T-cell Lymphoma. Am J Clin Dermatol 2018;19:805-19. [Crossref] [PubMed]
- Kim SJ, Kim JH, Ki CS, et al. Epstein-Barr virus reactivation in extranodal natural killer/T-cell lymphoma patients: a previously unrecognized serious adverse event in a pilot study with romidepsin. Ann Oncol 2016;27:508-13. [Crossref] [PubMed]
- Küçük C, Hu X, Jiang B, et al. Global promoter methylation analysis reveals novel candidate tumor suppressor genes in natural killer cell lymphoma. Clin Cancer Res 2015;21:1699-711. [Crossref] [PubMed]
- Lemonnier F, Dupuis J, Sujobert P, et al. Treatment with 5-azacytidine induces a sustained response in patients with angioimmunoblastic T-cell lymphoma. Blood 2018;132:2305-9. [Crossref] [PubMed]
- Ruan J, Moskowitz AJ, Mehta-Shah N, et al. Multi-Center Phase II Study of Oral Azacitidine (CC-486) Plus CHOP As Initial Treatment for Peripheral T-Cell Lymphoma (PTCL). 62nd ASH Annual Meeting and Exposition; 2020 2020/12/05: ASH.
- Dalton T, Doubrovina E, Pankov D, et al. Epigenetic reprogramming sensitizes immunologically silent EBV+ lymphomas to virus-directed immunotherapy. Blood 2020;135:1870-81. [Crossref] [PubMed]
- Maschalidi S, Sepulveda FE, Garrigue A, et al. Therapeutic effect of JAK1/2 blockade on the manifestations of hemophagocytic lymphohistiocytosis in mice. Blood 2016;128:60-71. [Crossref] [PubMed]
- Trantham T, Auten J, Muluneh B, et al. Ruxolitinib for the treatment of lymphoma-associated hemophagocytic lymphohistiocytosis: A cautionary tale. J Oncol Pharm Pract 2020;26:1005-8. [Crossref] [PubMed]
- Zhou L, Liu Y, Wen Z, et al. Ruxolitinib combined with doxorubicin, etoposide, and dexamethasone for the treatment of the lymphoma-associated hemophagocytic syndrome. J Cancer Res Clin Oncol 2020;146:3063-74. [Crossref] [PubMed]
- Onozawa E, Shibayama H, Takada H, et al. STAT3 is constitutively activated in chronic active Epstein-Barr virus infection and can be a therapeutic target. Oncotarget 2018;9:31077-89. [Crossref] [PubMed]
- Song TL, Nairismägi ML, Laurensia Y, et al. Oncogenic activation of the STAT3 pathway drives PD-L1 expression in natural killer/T-cell lymphoma. Blood 2018;132:1146-58. [Crossref] [PubMed]
- Ando S, Kawada JI, Watanabe T, et al. Tofacitinib induces G1 cell-cycle arrest and inhibits tumor growth in Epstein-Barr virus-associated T and natural killer cell lymphoma cells. Oncotarget 2016;7:76793-805. [Crossref] [PubMed]
- Nairismägi M. Oncogenic activation of JAK3-STAT signaling confers clinical sensitivity to PRN371, a novel selective and potent JAK3 inhibitor, in natural killer/T-cell lymphoma. Leukemia 2018;32:1147-56. [Crossref] [PubMed]
- Perrine SP, Hermine O, Small T, et al. A phase 1/2 trial of arginine butyrate and ganciclovir in patients with Epstein-Barr virus-associated lymphoid malignancies. Blood 2007;109:2571-8. [Crossref] [PubMed]
Cite this article as: Glover A, Shannon-Lowe C. From pathobiology to targeted treatment in Epstein Barr virus related T cell and Natural Killer cell lymphoproliferative diseases. Ann Lymphoma 2021;5:31.