State of the art in the diagnosis, biology and treatment of primary mediastinal B-cell lymphoma: a review
Introduction
Primary mediastinal B-cell lymphoma (PMBL) is a rare entity that is characterized in the WHO classification as a mature aggressive large B-cell lymphoma (LBCL) of presumed thymic B-cell origin that mostly invades the mediastinum. Many specific clinical and biological characteristics distinguish this disease from other lymphomas (1). Accounting for nearly 2–4% of newly diagnosed non-Hodgkin lymphomas (NHL), it mostly affects young female patients (median age 37 years) (2) and often manifests as a large and compressive anterior mediastinal mass. Nearly all studies have shown very good prognosis for PMBL, with a survival rate exceeding 80% at 5 years (3-5); this is a singular aspect of PMBL. Although initially classified as a subgroup of diffuse large B-cell lymphoma (DLBCL), it is now well established that PMBL presents some features that overlap with those of classical Hodgkin lymphoma (cHL), particularly nodular sclerosis cHL (NS-cHL). The two entities show distinct histopathological features but may share common clinical features (young patients with a large mediastinal mass eventually “bulky” ≥10 cm) as well as common biological and molecular characteristics (6). Indeed, recurrent genomic rearrangements (isochromosome 9p) and copy number alterations (I) of the 9p24.1 locus, which contains genes encoding the immune checkpoint ligand genes PDL1 and PDL2, lead to the overexpression of these genes by tumor cells (7-10). The binding of PDL1 or PDL2 to the PD1 receptor expressed by reactive T cells in the microenvironment increases the inhibitory signal mediated by PD1, resulting in a decrease in T-cell cytotoxic activity and providing an immune escape pathway to lymphoma cells. A second similarity is that three major molecular pathways, i.e., JAK-STAT, NF-kB and PD1/PDL1, are the key lymphomagenesis drivers in both PMBL (11,12) and NS-cHL (13-15).
The frontline combination of rituximab with anthracycline-based chemotherapy has greatly improved the outcome of both PMBL patients and those with other B-cell aggressive lymphomas. Nevertheless, some debate remains in the community regarding the superiority of dose-dense or dose-intensified regimens versus standard rituximab, cyclophosphamide, doxorubicin, vincristine, prednisone (R-CHOP) (16-18). The roles of autologous stem cell transplantation (ASCT) and consolidation radiotherapy (CRT) are also still a topic of discussion. In addition, fluorodeoxyglucose (FDG) positron emission tomography (PET) monitoring for treatment response assessment is another issue in PMBL management due to the finding of frequent false-positive exams with inflammatory mediastinal residual uptake without evidence of active disease within the patient’s biopsy samples (19,20). If 80–85% of PMBL patients can be cured after first-line therapy, refractory or relapsing (R/R) PMBL patients represent a real unmet medical need (21), and disease progression usually occurs very early, reflecting the chemoresistance of the disease.
In this review, we will summarize major data regarding the diagnosis, metabolic imaging, first-line treatment and relapse treatment of PMBL. We will also synthetize available emerging data that support the use of liquid biopsy in PMBL patient monitoring.
Clinical presentation and issues at diagnosis
PMBL typically occurs in young patients (median age 37 years) and presents as a localized large anterior mediastinal mass. There is a clear but unexplained female predominance (sex ratio female/male: 2:1), and the large majority of patients develop “bulky” disease, defined as a tumor mass larger than 10 cm in diameter. Approximately 80% of PMBL presents as localized stage I–II disease (22). Contrary to DLBCL, extranodal invasion and bone marrow involvement are uncommon, but contiguous regional organ involvement (lungs, pericardium, pleura) is a frequent finding (23). There is usually no leukemic phase. Of note, a posterior mediastinal mass may suggest diagnosis of mediastinal Gray-Zone lymphoma (MGZL) or alternative etiologies rather than PMBL. In addition, PMBL is frequently extranodal when outside the mediastinum which can help distinguish it from MGZL that is commonly nodal when outside the mediastinum (24).
Clinical symptoms at diagnosis are related to the mediastinal mass, frequently as a superior vena cava syndrome (SCVS), resulting in thrombotic complications in 30–40% of patients who present with a bulky anterior mediastinal mass at diagnosis, which may affect overall survival (OS) (25). This complication warrants the prompt use of steroids in cases of SVCS to reduce the tumor mass that is causing the obstruction (26,27). However, systematic anti-thrombotic therapy is not recommended in case of malignant SVCS. Indeed, PMBL patients can frequently have vascular compression on imaging without true thrombosis and routine use of anticoagulation can complicate treatment which can lead to thrombocytopenia and bleeding risk (28). In addition, very recent international clinical practice guidelines for the treatment and prophylaxis of venous thromboembolism in patients with cancer does not mention the use of anti-thrombotic therapy in patients with PMBL and/or SVCS (29). The diagnosis of PMBL is often made in an emergency context due to the frequently severe clinical presentation and the symptoms related to the tumor mass and requires mediastinoscopy, an invasive thoracic surgical procedure in which the mediastinum is examined and tissue is sampled for histological analysis. This procedure is performed under general anesthesia and often requires admission of the patient to the intensive care unit when he or she presents with SCVS. The risks of mediastinoscopy include bleeding, infection, temporary or permanent paralysis of the laryngeal nerve, pneumothorax, subcutaneous emphysema and esophageal or tracheal perforation. Mediastinoscopy may be difficult in cases of adherences secondary to previous local surgery (previous mediastinoscopy, neck or cervical spine surgery) or health conditions that prevent proper positioning of the neck during the procedure. Of note, tissue sampling can also be performed by needle biopsy under scanner guidance, but this leads to other concerns since the material may be of insufficient quality and quantity for optimal histopathological diagnosis. This technical difficulty is a common routine issue that impacts the initial management of patients.
Finally, the diagnosis of PMBL is a clinicopathological diagnosis based on the typical clinical presentation of the disease (young patients, female predominance, large and isolated anterior mediastinal mass) combined with histopathological and biological features.
Histology and morphological features of PMBL
The histopathological diagnosis of PMBL is based on a broad spectrum of morphological features combined with the typical immunophenotype of the disease and correlation with clinical data. The morphology is characterized by a diffuse growth pattern within a variable degree of fibrosis, resulting in a crush appearance and/or a compartmentalization of tumor cells (1).
The neoplastic cells present in PMBL are usually medium to large in size and have round, multilobated or pleomorphic nuclei and cytoplasm that varies in appearance (pale, slightly basophilic or clear). “Clear cell morphology”, which is related to a hydropic change in the cytoplasm, is a common feature of PMBL cases (30). The presence of Reed-Sternberg-like cells may suggest the possible presence of cHL, especially in cases in which there are bands of sclerotic tissue and immune cell infiltration, although these features are present to a lesser extent in PMBL (1). No correlation between the different morphological patterns noted in PMBL and outcome was found in a large retrospective study performed before the rituximab era (30).
The immunophenotype of PMBL lymphoma cells is characterized by the expression of B-cell-lineage surface and cytoplasmic markers such as CD19, CD20, CD22 and CD79a and transcription factors such as PAX5, OCT2, and BOB1 (23,31-35). CD30 is expressed in >80% of cases but shows weak and heterogeneous staining (32), unlike in cHL, and CD15 expression is frequently negative (36) but up to 6% of PMBL patients can show CD15 expression (24). CD23 is expressed in 70% of cases (31,37,38), and PDL1/PDL2 is expressed in 50–70% of cases (39). There is no EBV infection (1). BCL2 is positive in 55–80% if cases and BCL6 is positive in 45–100% of cases (32,40). MUM1/IRF4 is positive in 75–90% of cases (41). MAL antigen expression has been described as a distinguishing marker of aggressive B-cell lymphomas and may help identify PMBL in difficult cases (42,43), but the use of anti-MAL antibodies in routine practice remains limited due to technical difficulties and difficulties in interpretation. Consistent with an immune escape phenotype, PMBL cells commonly lack HLA class I and/or class II molecules. The immunophenotype of PMBL is summarized in Table 1.
Table 1
Epitope/antigen | Lymphoma cells | Comments | Main reference(s) |
---|---|---|---|
CD3 | Negative | Nests of CD3+ or CD5+ lymphocytes are present in the microenvironment, with a perivascular distribution | (23,33) |
CD5 | Negative | ||
CD20 | Positive | All large cells typically express CD20 on the membrane | (31,32,34,35) |
CD23 | Positive in 70% of cases | CD23 is a transmembrane glycoprotein predominantly expressed in mature IgD+ B lymphocytes and follicular dendritic cells | (37,38) |
CD10 | Usually negative | CD10 positivity described in 8–32% of cases | (31,32) |
CD15 | Frequently negative | Myeloid/monocyte marker positive in 80% of cHL | (36) |
CD30 | Positive | Typically weak or moderate and heterogeneous expression in PMBL | (32) |
BCL2 | Positive in 55–80% of cases | Expression is inhibited in reactive germinal centers | (40) |
BCL6 | Positive in 45–100% of cases | Mainly expressed in germinal center cells | (40) |
MUM1/IRF4 | Positive in 75–90% of cases | Expressed in late plasma cell-directed stages of B-cell differentiation | (41) |
PDL1/PDL2 | Positive in 50–80% of cases | Staining also noted in macrophages, histiocytes and dendritic cells, localized primarily to the cytoplasm and of weaker intensity in microenvironment | (39) |
PMBL, primary mediastinal B-cell lymphoma; cHL, classical Hodgkin lymphoma.
PMBL should not be confounded with MGZL, the new appellation for what was formerly designated “B-cell lymphoma, unclassifiable, with features intermediate between DLBCL and cHL” (44). The diagnostic criteria for MGZL notably include high tumor cell density and strong expression of ≥2 B-cell markers on immunohistochemistry (IHC) (24,45). However, cases of composite and sequential lymphoma that show characteristics between those of cHL and PMBL occur very rarely, and this argues for major tumor cell plasticity in MGZL (46). With regards to differential diagnoses of PMBL, we should highlight the works of the Leukemia/Lymphoma Molecular Profiling Project (LLMPP) and French gene expression assay which are developed with the goal to implement gene expression profiling (GEP) as diagnostic assays to distinguish PMBL from DLBCL, cHL and MGZL. First, in 2003, the LLMPP used Lymphochip DNA microarrays and a 46 gene Bayesian predictor to study molecular diagnosis (based on GEP signature) and established a robust molecular predictor that precisely distinguishes PMBL from DLCBL (6). Then, in 2015, the LLMPP applied this PMBL predictor to identify 24 PMBL cases in a large series of DLBCL cases, and 6 of these 24 cases had no evidence of mediastinal disease and would have been underdiagnosed by clinicians and pathologists without this tool (47). In 2017, a reliable, rapid and cost-effective method based on reverse transcriptase multiplex ligation-dependent probe amplification (RT-MLPA) was developed by a French team. The authors built a PMBL signature with CD30, MAL and CD23 genes (overexpressed in these lymphoma) and demonstrated that this RT-MLPA tool was useful to discriminate PMBL from DLBCL using a Bayesian predictor (48). Finally, in 2020, the same French team combined an extended GEP panel (with more than 130 genetic markers, including 12 PMBL markers) and machine learning in an accurate pan-B-NHL predictor (LymphoSign test, Genexpath®, Rouen, France), able to identify the PMBL diagnosis (49). These French and LLMPP GEP tools that require RNA extraction from FFPE samples may be proposed as a complement to conventional histology to help pathologists to identify PMBL cases. To conclude, accurate identification of PMBL patients and selection for outcomes research and clinical trials (CTs) within aggressive B-cell lymphoma is important, and lack of such assays has hampered progress and execution of dedicated phase III PMBL trials. An effort should be made to implement the routine use of such GEP tools.
Biology of PMBL: distinct cell-of-origin, immune escape profile and strong similarities to cHL
The putative origin of PMBL cells is a thymic medullary, asteroid, activation-induced cytidine deaminase (AID)-positive B-cell (50). Thymic B cells display a CD20+CD21− and IgM+ but IgG− immunophenotype (51), reside at the corticomedullary junction of the thymus and are estimated to represent approximately 1% of total thymic cells (52). Most thymic B cells are naïve B cells, and they are distinct from peripheral blood B cells (53). In addition, they display a phenotype that mimics antigen-presenting cells, with overexpression of CD86 and downregulation of MHC II expression. Furthermore, they strikingly express autoimmune regulator (AIRE), an important transcriptional regulator that is not expressed by peripheral B cells. Though the presence of B cells in the thymus has been well established, their origin remains a matter of debate. Three decades ago, it was demonstrated in mice that thymic B cells emerged outside of the bone marrow with the finding that mature thymic B cells are present at embryonic day 18, that is earlier than B cell differentiation start in the bone marrow (54,55). Unlike most B cells that differentiate in the bone marrow, thymic B cells differentiate within the thymus from early thymic precursors (56). In addition, several robust experiments established that peripheral B cells are almost all unable to migrate into the thymus when splenocytes are injected intravenously, which supports the idea that peripheral B-cell cannot recirculate to the thymus from the periphery (57-59). Taken together, these findings do not support the hypothesis of peripheral B-cell homing (60) to the thymus, so the main theory is the existence of an intrathymic pathway for B cell onset and maturation (61). However, it is challenging to draw clear-cut conclusions through the origin and persistence over time of thymic B-cells, as our understanding mainly derived from experiments in young mice. Furthermore, there are few robust biological studies of thymic B cells and PMBL lymphomagenesis (33,62,63). Of note, the typical high level of BCL6 expression in PMBL cases, in addition to the high mutational burden, partially contradicts the hypothesis of a naïve B-cell of origin (COO), and this is confirmed by the distinct landscape of somatic alterations compared with other germinal-center (GC) B-cell lymphomas [follicular lymphoma (FL) and DLBCL], implying a specific and separate COO (64).
Recently, Mottok et al. analyzed a series of 95 PMBL tumors by whole-exome sequencing (WES) and demonstrated that SOCS1 (suppressor of cytokine signaling 1) is the most commonly mutated gene (~60%). Interestingly, PTPN1 mutations have been described in approximately 20% of PMBL (65); these mutations result in decreased expression of the gene, which encodes the PTP1B protein, as detected by IHC. These mutations result in increased phosphorylation of components of the JAK-STAT pathway, a phenomenon that also occurs in HL cell lines silenced for PTPN1, suggesting that the JAK-STAT pathway may be implicated in PMBL lymphomagenesis. Furthermore, in ~25% of PMBL cases, gain-of-function IL4R mutations (66) that generate constitutive activation of the JAK-STAT pathway in PMBL cell lines were described. These cells secrete high amounts of CCL17 in vitro and in vivo and thus may cooperate in T-cell engagement. Recently, an abstract by Noerenberg et al. (67) that was presented at EHA 2022 reported the results of a large biological study of 486 PMBL samples using whole-genome/WES and targeted sequencing. The authors confirmed the landscape of established PMBL driver genes, finding a mutational burden of 5 mutations/Mb and reporting that the 10 most frequently mutated driver genes were SOCS1 (86%), B2M (67%), ITPKB (64%), ACTB (58%), STAT6 (58%), IGLL5 (56%), TNFAIP3 (53%), NFKBIE (49%), GNA13 (47%), and ZNF217 (36%). Several somatic variants had an impact on outcome: patients with mutations in CD58 displayed lower survival rates than patients without such mutations [progression-free survival (PFS): hazard ratio (HR) =2.96; P<0.001; OS: HR =2.55; P=0.006], while patients with mutations in DUSP2 had a favorable prognosis (PFS: HR =0.28; P=0.002; OS: HR =0.15; P=0.011) (67). These correlates with clinical outcome are a great step forward in a more accurate understanding of PMBL. Table 2 provides a summary of the most common recurring somatic alterations in PMBL (11,67-72).
Table 2
Gene | Prevalence of somatic mutations | Pathway/role (source: GeneCards database) | Main reference(s) |
---|---|---|---|
SOCS1 | 45–86% | Negative regulation of cytokines JAK/STAT pathway | (67,68) |
B2M | 40–67% | Presentation of peptide antigens to the immune system | (11,67) |
ITPKB | 8.9–64% | Cellular signaling | (67,69) |
ACTB | 56% | Cytoplasmic cytoskeleton, regulation of gene transcription, repair of damaged DNA | (67) |
STAT6 | 36–58% | Signal transduction and activation of transcription. IL4/interleukin-4- and IL3/interleukin-3-mediated signaling | (67,70) |
IGLL5 | 56% | Immunoglobulin lambda-like polypeptides | (67) |
TNFAIP3 | 9.5–53% | Cytokine-mediated immune and inflammatory responses; NF-kappa B pathway | (67,69) |
NFKBIE | 22.7–49% | NF-kappa B pathway; inhibits binding of NF-kappa B p50-p65 and p50-c-Rel complexes to DNA | (67,71) |
GNA13 | 7.6–47% | Promotes tumor cell invasion and metastasis by activating the RhoA/ROCK signaling pathway | (67,69) |
ZNF217 | 36% | Promotes cell proliferation and antagonizes cell death | (67) |
IL4R | 24.2% | Inhibits IL4-mediated cell proliferation and IL5 upregulation by T cells | (66) |
XPO1 | 24% | Nuclear export; control of several cellular processes by controlling the localization of cyclin B, MPAK, and MAPKAP kinase 2 | (72) |
PTPN1 | 22% | Cell growth, differentiation, mitotic cycle, and oncogenic transformation | (65) |
PMBL, primary mediastinal B-cell lymphoma; JAK/STAT, Janus kinase/signal transducers and activators of transcription; MAPKAP, MAP kinase-activated protein kinase.
Immunoglobulin genes are typically rearranged in association with immunoglobulin class switching and a high load of V(D)J somatic hypermutation without ongoing somatic mutational activity, suggesting that the B-cell progenitor that gives rise to PMBL is altered by the GC environment during lymphomagenesis (73). However, the absence of ongoing somatic mutation remains unclear in PMBL, although Popov et al. (74) suggested that the accessibility of target sequences is a major limiting factor for AID-dependent somatic gene diversification in PMBL. A lack of surface and cytoplasmic immunoglobulin protein is also a singular feature of PMBL (36,73) but has also been frequently described in cHL (75).
Twenty years ago, the application of GEP technology using DNA microarrays to frozen PMBL tumors and DLBCL tumors revealed divergent molecular signatures of PMBL and DLBCL (6). Remarkably, the researchers isolated a unique PMBL signature. In detail, the JAK2, PDL1, PDL2 and SMARCA2 genes, all of which are located on chromosome band 9p24, are expressed at considerably higher levels in PMBL than in DLBCL, consistent with other reports (54) showing recurrent gains in 9p in PMBL (76). Furthermore, PDL2 was the gene that best discriminated between PMBL and DLBCL. The microarray transcriptional profile of frozen PMBL biopsies was similar to that of cHL but distinct from DLBCL transcriptomes (77). Although PMBL and cHL cell lines appeared to be closely related, there were some discrepancies: numerous B-cell lineage markers (CD19, CD20, CD22, and CD79) were not detected in cHL cell lines but were detected in PMBL cell lines (78-80). IHC staining for PDL1 and PDL2 was positive in approximately half of PMBL cases versus 95% (PDL1) and 23% (PDL2) of cHL cases (81). Using genome-wide DNA copy number analysis (7), Shipp’s group demonstrated amplification of 9p24 in 100% of cHL and PMBL cell lines compared to only 22% of DLBCL cell lines. These 9p gains were also detected using laser-capture microdissected Hodgkin Reed-Sternberg cells and PMBL biopsies and were found by IHC to correlate with PDL1/2 protein overexpression in primary tumors (7). PDL1/2 rearrangements (including 9p24.1 break-apart and 9p24.1 amplification) were more frequently detected by fluorescent in situ hybridization (FISH) analysis in PMBL (20%) than in any other type of lymphoma (DLBCL, follicular lymphoma, primary testicular DLBCL, and nodular-lymphocyte predominant HL) (10) and correlated with overexpression of PDL1/2 transcripts.
Furthermore, several teams reported increased expression of genes associated with the JAK-STAT and NF-KB pathways in PMBL (77,82,83). Indeed, 9p24 rearrangements also encompass JAK2, the overexpression of which results in increased protein activity, further inducing PD-1 ligand transcription. Adding further support to the idea that PMBL exhibits an “immune escape” profile and shows similarities with cHL, Steidl’s group detected rearrangements of the CIITA gene, which encodes the major histocompatibility complex (MHC) class II transactivator, in 38% of PMBL cases by FISH analysis; such rearrangements were also detected in 15% of cHL cases and in only 3% of DLBCL cases (84). This rearrangement was initially discovered by RNA sequencing of cHL cell lines and is associated with a marked reduction in CIITA protein expression. Cases with CIITA rearrangements involving PDL1 and PDL2 as partner genes displayed higher PDL1 and PDL2 overexpression than cases with other partner genes, indicating that the CIITA promoter drives the expression of these two immune checkpoint inhibitors, resulting in the inhibition of T-cell activation in vitro (84). In accordance with the PMBL immune privilege phenotype, patients who displayed CIITA rearrangements had inferior outcomes (10-year disease-specific survival 63.6% compared with 85.0% in patients who did not display such rearrangements; P=0.044) (84). In another study, transduction of a DLBCL lymphoma cell line with a lentiviral vector containing the CIITA fusion detected by RNAseq led to decreased MHC II expression. Furthermore, lower CD4 and CD8 T-cell infiltration of PMBL tumor samples measured by IHC staining was also linked to downregulation of MHC class II antigen expression (85). There was also a loss of expression of MHC class I antigen in the cell membranes of PMBL tumor cells, as described for cHL (86). Finally, a very recent biological study shows that loss of heterozygosity (LOH) appears to be a novel and distinctive feature of PMBL. In that study, Tuveri et al. demonstrated that large-scale copy-neutral LOH participates in the molecular pathogenesis of PMBL and that LOH events that cluster in the chromosomal regions 6p (60%), 15 (37.2%) and 17q lead to homozygosity conversion of well-known PMBL driver genes (87). This mechanism was found to frequently co-occur with homozygous mutations in MHCI (6p21), B2M (15q15), and GNA13 (17q23) (87).
Taken together, studies of PMBL cases have highlighted the biological complexity of this tumor and contributed to building the concept that PMBL tumors possess “immune privilege” or an immune escape profile that results from specific epigenetic, genetic and molecular alterations that represent a main driver of PMBL lymphomagenesis. All of these features distinguish PMBL from DLBCL, MGZL and cHL.
Extramediastinal PMBL
Several teams have reported that, notably, “PMBL-like” lymphomas can be identified at nonmediastinal sites. Indeed, cases of DLBCL with features of PMBL but without detectable mediastinal involvement have been reported, and using GEP methods, a PMBL signature may be identified in those cases (47). More recently, Duns et al. further delineated these particular lymphomas using the PMBL GEP signature in a cohort of 325 de novo DLBCL patients. The authors observed that 16 of these cases express MAL and CD23 and harbor SOCS1, IL4R, ITPKB, and STAT6 mutations, all of which are typical PMBL features. In addition, in contrast to bona fide PMBL, the patients were older and never presented with pleural involvement (88). This subset of “molecular PMBL” appears to represent a distinct subgroup of DLBCLs that share molecular features with bona fide PMBL, but further research is warranted to gain a better understanding of the underlying lymphomagenesis process that generates these very uncommon extramediastinal PMBLs and to determine whether a specific therapeutic strategy should be applied in such cases.
The central role of PET in PMBL staging and evaluation
FDG-PET is the current gold standard for extension at diagnosis and response assessment in PMBL. Its interpretation is based on the Deauville score (89,90) and Lugano 2014 criteria (91). Obtaining a complete metabolic response (CMR) at the end of treatment represents a remarkable outcome. Indeed, in the landmark IELSG-26 study (92), the 5-year PFS for PMBL with positive [as defined by International Harmonization Project (IHP) criteria with residual uptake > mediastinum, (93)] and negative (i.e., Deauville score ≤2, residual uptake ≤ mediastinum) end-of-treatment PET was 68% vs. 99%, respectively (P<0.001). Nevertheless, PET assessment of PMBL after treatment is difficult given that a slightly hypermetabolic residual mediastinal mass often persists during the interim and at the end of treatment without the systematic presence of lymphoma cells on rebiopsy (94). After the publication of the Lugano classification (95) and the results of the IELSG-26 study, the definition of CMR was changed from Deauville score 2 to Deauville score 3 so that the reference threshold used to define CMR was changed from the mediastinal blood pool activity to the activity in normal liver (96).
In a remarkable study, Ceriani et al. established that PET has a high negative predictive value at the end of treatment (i.e., all the patients obtaining a CMR defined as Deauville score ≤3 remained progression-free at 5 years) but a poor positive predictive value (97). These findings are often described in cases that present large bulky masses with a major fibrotic component at diagnosis (98). Indeed, recurrent persisting PET positivity without disease progression is a particular feature of PMBL. Vassilakopoulos et al. described a cohort of 182 patients treated with standard R-CHOP; 16% of these patients had Deauville scores of 4 at the end of treatment and a 5-year failure-free probability of 82%, compared to 92% for patients with Deauville scores of 3 (99). The same group also noted that many patients who displayed IHP positive PET after R-CHOP continued to be positive after CRT and remained in long-term remission without further treatment and were thus considered “false-positives” (100). An end-of-treatment PET standardized uptake value (SUV) ≤5.4 may also delineate a subgroup of patients with favorable outcomes (101), but this isolated finding requires further validation.
Recently, the lymphoma study association (LYSA) group reported a very large retrospective cohort of 313 PMBL patients, of whom 26 had Deauville scores of 4 (considered positive) at the end of treatment and displayed excellent outcomes, notably a 3-year OS rate of ~90% (18). In this study, approximately 23% of PMBL patients received ASCT, 10-fold higher than the percentage of patients with DLBCL in the GAINED trial (102). This high rate of ASCT may have in part been influenced by false-positive interim PET exams, given that disease control at the end of induction appeared comparable. In the report by Ceriani et al. based on the IELSG-26 study, patients with Deauville scores of 4 at the end of treatment had also better outcomes than those with Deauville scores of 5 (5-year time-to-progression: 87% vs. 33%, P=0.0002) (103). That being said, we should clearly advise against salvage chemotherapy and/or consolidation ASCT in chemosensitive patients based solely on PET positivity (based on Lugano criteria) at the end of treatment in the absence of histopathologically proven progressive or multifocal disease (20,99,100). In the report by Melani et al., up to 50% of Deauville score 5 patients were considered to have false-positive exams. Therefore, in the case of residual uptake in Deauville score 4–5 patients at the end of treatment, we recommend a “watch and wait” attitude with serial imaging surveillance if there are no other clinical indicators of disease progression. Lazarovici et al. (94) described the low predictive value of positive interim PET assessed by the Deauville score in PMBL patients. With that in mind, new tools are required to improve the evaluation of therapeutic response in PMBL. Maximum standardized uptake value (SUVmax) reduction between baseline (PET 0) and PET after 2 and 4 cycles of chemotherapy (ΔSUVmax PET 0–2: cutoff ≤66% or >66%, and ΔSUVmax PET 0–4: cutoff ≤70% or >70%), as described in the GAINED trial, appears to be an interesting alternative for evaluation of the therapeutic disease response (102). In the retrospective LYSA study, the authors observed that ΔSUVmax PET 0–4 ≤70% was associated with unfavorable outcome (18). This tool is useful in guiding consolidation decisions regarding the treatment of DLBCL patients and appears to be more accurate than visual analysis (104,105). However, a dedicated prospective study that tests this hypothesis in the PMBL setting would be of major interest.
Prognostic features at diagnosis
The international prognostic index (IPI) score widely used in DLBCL prognostic assessment is probably not suitable for accurately assessing the prognosis of patients with PMBL. Indeed, the combination of extranodal (EN) involvement and elevated lactate dehydrogenase (LDH) ≥2 times the upper limit of normal (ULN) or bulky disease appears more relevant to define a “high risk” subgroup (13–27% of patients) with an approximately 20% rate of lymphoma-related mortality (106). Patients without any of these risk factors represent, in contrast, an ultralow risk subgroup with 11% risk of failure and only 1% to 4% 5-year lymphoma-related mortality (106). In addition, the absence of B symptoms at diagnosis appears to be a favorable prognostic factor (107). Pleural or pericardial involvement was also a predictive factor for PFS, but the proposed PMBL-dedicated IPI (PMBIPI), which integrated those factors (16), remains rarely used because of the lack of independent validation.
Regarding biological factors, Zhou et al. reported that MUM1 negativity and lower lymphocyte-to-monocyte ratio were associated with inferior OS and PFS, respectively (101). Conversely, Bledsoe et al. observed that low PDL1 (P=0.011) and high MUM1 (P=0.065) led to inferior PFS. In another report, MUM1-positive cases had very unfavorable outcomes (108). The variations in the characteristics of the patients included in the different studies, the variety of treatments used and the well-known limits of IHC reproducibility preclude drawing definitive conclusions regarding the applicability of these biological prognostic factors in routine practice.
Regarding PET features, the LYSA group demonstrated that a baseline total metabolic tumor volume (TMTV) ≥360 cm3 was associated with unfavorable prognosis independent of treatment. Liu et al. reported that total lesion glycolysis (TLG) ≥2,500 at baseline correlated with worse PFS (P=0.023) (107). In the IELSG26 study, the combination of baseline TLG and end-of-treatment Deauville score showed a better positive predictive value than TLG alone, and patients with TLG >5,814 and interim Deauville scores of 4–5 showed clearly inferior outcomes (109).
Finally, central nervous system relapses are a rare (~3%) but severe event that may occur during the course of PMBL disease (18), and there is currently no dedicated predictive score that can be used to anticipate this risk in PMBL patients. Central nervous system (CNS) IPI is routinely used in PMBL management, by analogy to DLBCL, but it has not been specifically assessed in large PMBL studies (110) and may not be helpful in evaluating the risk of CNS recurrence in this disease (111). Of note, there is no recommendation regarding CNS prophylaxis in PMBL.
Frontline treatments: the role of dose intensity
The first-line regimens used to treat PMBL patients vary across Western countries, and the best combination remains debated. Standard immunochemotherapy includes R-CHOP (5,103) delivered every 14 (RCHOP14) or 21 days (RCHOP21), dose-dense R-ACVBP with PET-driven consolidation (3,102,112) or DA-EPOCH-R (4,113). Mediastinal CRT was historically administered after chemotherapy but is currently generally reconsidered given its toxicity in this young patient population (114,115). Table 3 outlines the effectiveness of the main first-line treatment schemes. Of note, before the rituximab era, Massoud et al. reported better OS and PFS after intensified CHOP (namely, “ACVBP”) compared to CHOP21 in PMBL (3), suggesting that “dose intensity” may be a prognostic factor in PMBL. In the rituximab era, the well-known study conducted by Dunleavy et al. also demonstrated that treatment with an intensive DA-EPOCH-R regimen obviated the need for CRT in chemosensitive PMBL patients (4). It is interesting to note that the use of DA-EPOCH-R has greatly increased (13% vs. 59%, P<0.001) since the publication of Dunleavy et al. in 2013, with a concomitant marked decrease in the use of CRT (13% vs. 59%, P<0.001) (116). Complete response (CR) rates appeared higher after DA-EPOCH-R in this series than after R-CHOP21 (84% vs. 70%, P=0.046). However, the DA-EPOCH-R regimen has not come into use in all hematology centers, probably due to its complexity and its hematological toxicity compared to RCHOP14/21. Indeed, R-CHOP and DA-EPOCH-R have a similar backbone of prednisone, cyclophosphamide, vincristine, doxorubicin and rituximab but besides the addition of etoposide, the procedure to administer them strongly differs. To receive DA-EPOCH-R, patients need to be hospitalized, because doxorubicin, etoposide, and vincristine are infused over 96 hours and cyclophosphamide is given after completion of the 96-hour infusion (118). These repeated hospitalizations may be associated with financial toxicity in many countries. In contrast, R-CHOP14 and R-CHOP21 are injected in an outpatient setting as bolus chemotherapy. In addition, DA-EPOCH-R is designated to enhance dose intensity by adjusting the doses of etoposide, cyclophosphamide, and doxorubicin based on the platelet and neutrophil nadirs of each cycle (119), adding to the complexity of the DA-EPOCH-R regimen management.
Table 3
Ref. | No. of patients | Study design | IPI | First-line regimen | % of patients receiving CRT | % of patients receiving upfront consolidation ASCT | Response rate | EFS/PFS/TTP | OS |
---|---|---|---|---|---|---|---|---|---|
(4) | 51 | Prospective phase II study | NA | DA-EPOCH-R | 3.9% | No | CMR =94.1% | 5-year EFS: 93% | 5-year OS: 97% |
(16) | 240 | Retrospective | IPI 0–1: 52%; IPI 2: 25%; IPI 3: 13%; IPI 4–5: 10% | R-CHOP: n=187; CHOP: n=44; DA-EPOCH-R: n=9 | All regimens: 42%; R-CHOP group: 34% | R-CHOP: 90%; CHOP: 67%; DA-EPOCH-R: 100% | R-CHOP: CR =64.1% | R-CHOP + IFRT: 4-year PFS: 85% | R-CHOP + IFRT: 4-year OS: 100% |
(5) | 50 | Prospective subgroup analysis of UK NCRI R-CHOP 14 v 21 trial | NA | R-CHOP14: n=22; R-CHOP21: n=28 | 58% | NA | ORR 92%, CR 43% | 5-year PFS: 80% | 5-year OS: 84% |
(116) | 132 | Retrospective | IPI 0–1: 66%; IPI 2–3: 31%; IPI 4–5: 3% | R-CHOP21: n=56; DA-EPOCH-R: n=76 | R-CHOP21 group: 59%; DA-EPOCH-R group: 13% | R-CHOP21: 3.6%; DA-EPOCH-R: 1.3% | R-CHOP21: ORR 80.3%, CR 69.6%; DA-EPOCH-R: ORR 92.1%, CR 84.2% | R-CHOP21: 2-year PFS 73%; DA-EPOCH-R: 2-year PFS 85% | R-CHOP21: 2-year OS 89%; DA-EPOCH-R: 2-year OS 91% |
(113) | 69 | Prospective | NA | DA-EPOCH-R: n=36; R-CHOP21: n=33 | NA | NA | DA-EPOCH-R: ORR 97.7%, CR 85%; R-CHOP21: ORR 81.8%, CR 50% | DA-EPOCH-R: 5-year PFS 90.9%; R-CHOP21: 5-year PFS 64.1% | DA-EPOCH-R: 5-year OS 97.7%; R-CHOP21: 5-year OS 73.8% |
(103) | 159 | Retrospective | IPI 0–1: 36%; IPI 2–3: 47%; IPI 4–5: 14% | R-CHOP21: n=149; R-CHOP/R-ICE: n=8; DA-EPOCH-R: n=2 | 44% | No | CMR: 63% | R-CHOP21 5-year TTP: 79% | R-CHOP21 5-year OS: 88% |
(117) | 53 | Retrospective | IPI 0–2: 72%; IPI 3–5: 28% | R-CHOP21: n=21; DA-EPOCH-R: n=28 | R-CHOP21: 100%; DA-EPOCH-R: 59.3% | R-CHOP21: 40%; DA-EPOCH-R: 0% | R-CHOP21: 92% ORR, 60% CR; DA-EPOCH-R: 92.6% ORR, 70.4% CR | R-CHOP21: 1-year PFS 87%; R-CHOP21 + ASCT: 1-year PFS: 90%; DA-EPOCH-R: 1-year PFS 73.9% | R-CHOP21: 1-year OS 100%; R-CHOP21 + ASCT: 1-year OS: 100%; DA-EPOCH-R: 1-year OS: 92% |
(18) | 313 | Retrospective | IPI 0: 10.5%; IPI 1–2: 60.7%; IPI 3–5: 26.2% | R-ACVBP: n=180; R-CHOP14: n=76; R-CHOP21: n=57 | R-ACVBP: 2.2%; R-CHOP14: 14.5%; R-CHOP21: 3.5% | R-ACVBP: 25.6%; R-CHOP14: 31.6%; R-CHOP21: 1.8% | R-ACVBP: CMR =86.3%; R-CHOP14: CMR =86.8%; R-CHOP21: CMR =76.6% | R-ACVBP: 3-year PFS 89.4%; R-CHOP14: 3-year PFS 89.4%; R-CHOP21: 3-year PFS 74.7% | R-ACVBP: 3-year OS 92.4%; R-CHOP14: 3-year OS 100%; R-CHOP21: 3-year OS 87.5% |
(106) | 332 | Retrospective | IPI 0–1: 72.9%; IPI 2–5: 27.1% | R-CHOP14: n=30; R-CHOP21: n=302 | 72% | R-CHOP14: 3.3%; R-CHOP21: 0% | NA | Whole population: 5-year FFP: 77.6% | 5-year LSS: 89.1% |
PMBL, primary mediastinal B-cell lymphoma; IPI, international prognostic index; CRT, consolidation radiotherapy; ASCT, autologous stem cell transplantation; EFS, event-free survival; PFS, progression-free survival; TTP, time to progression; OS, overall survival; NA, not available; CMR, complete metabolic response rate; IFRT, involved field radiotherapy; ORR, overall response rate; CR, complete response; FFP, freedom from progression; LSS, lymphoma-specific survival.
In 2016, a randomized study of 69 PMBL Ukrainian patients reported that DA-EPOCH-R offers better efficacy than R-CHOP21 (113) despite its greater hematological toxicity. The rate of CRT use in the two arms was not reported. In contrast, Malenda et al. (117) demonstrated very similar excellent outcomes in 53 PMBL patients treated with first-line R-CHOP21 and DA-EPOCH-R. However, in this study, CRT was used for all patients in the R-CHOP21 group vs. 59.3% of patients in the DA-EPOCH-R group; and consolidative ASCT was performed for 40% of patients in the R-CHOP21 group vs. 0% in the DA-EPOCH-R group, which may likely explain the comparable efficacy between “DA-EPOCH-R alone” vs. “R-CHOP + CRT or R-CHOP + ASCT”. Another study reported by Held et al. in the form of an abstract (120) noted efficacy results comparable to those obtained using R-CHOP21 and R-CHOP14 in a subset analysis of 131 PMBL patients who participated in the UNFOLDER trial. The fact that only patients with age-adjusted IPI (aaIPI) =0 plus bulky disease or aaIPI =1 were included in either the CRT or the observation arms could somewhat explain these findings. Finally, the LYSA group recently reported a large series of 313 patients in France and Belgium who received first-line treatment with R-CHOP14, RCHOP21 or R-ACVBP (18): the 3-year PFS rates for the patients who received R-ACVBP, R-CHOP14 or R-CHOP21 were 89.4% [95% confidence interval (CI): 84.8–94.2%], 89.4% (95% CI: 82.7–96.6%) and 74.7% (95% CI: 64–87.1%) (P=0.018), respectively. Clearly, patients treated with R-CHOP21 had inferior outcomes compared to those who received dose-dense regimens (R-ACVBP and R-CHOP14). A recent study involving a Polish cohort of 124 patients with PMBL who underwent extended follow-up (median 9 years) also reported a high cure rate of more than 90% (5-year OS and PFS: 94% and 92%, respectively) after administration of an intensive immunochemotherapy protocol (GMALL/B-ALL/NHL2002) (121). Based on these findings, dose intensity appears to determine the excellent outcome of PMBL. However, Noerenberg et al. recently reported in an abstract at EHA 2022 that a subset of PMBL patients with DUSP2 mutations (n=80/319) had favorable outcomes with respect to CR rate, PFS and OS whether they received an R-CHOP-like regimen or intensified treatment, implying that there is no advantage of treatment intensification in this low-risk subgroup (67).
Finally, the role of and the indications for treatment intensification with ASCT as a frontline consolidation option remain unclear. In the prerituximab era, Cairoli et al. (122) observed in a very limited monocentric cohort of 15 patients that BEAM-ASCT after intensified VACOP-B induction followed by DHAP and/or CRT in cases of partial response offered durable complete remission in all but one patient (aa-IPI 2–3). However, this combination is toxic in this young population, and no long-term data are available, particularly regarding the second malignancy rate. Similar results were obtained by Hamlin et al. in a study that compared upfront ASCT and dose-dense anthracycline-based chemotherapy (123). Since the introduction of rituximab, the benefit of ASCT, as evaluated by interim PET response, remains debated due to the scarcity of prospective data in this setting and the absence of dedicated randomized studies (102,124,125). Indeed, PMBL patients are underrepresented in CTs, precluding the extension of the conclusions drawn in large phase III studies of DLBCL. Of note, the anti-CD30 antibody drug conjugate brentuximab-vedotin (BV) has shown promising efficacy in combination with R-CHP for the frontline treatment of PMBL patients who show at least 1% or higher expression of CD30 by IHC. In a small series of 30 patients, the authors demonstrated an objective response rate of 100% with 86% CR and 2-year PFS and OS rates of 85% and 100%, respectively (126). These preliminary data warrant validation in larger trials.
To conclude, there is no consensus regarding the optimal treatment strategy for newly diagnosed PMBL patients. However, in recent studies, excellent results were obtained for patients treated with dose-dense immunochemotherapy based on rituximab and anthracyclines with interim PET assessment (18,103). A proposed algorithm for treatment using R-CHOP14 or R-ACVBP induction and PET-driven consolidation is depicted in Figure 1. Given the favorable outcomes with intensive immunochemotherapy alone, consolidative therapeutic intensification with ASCT should no longer be considered standard of care in the frontline setting.
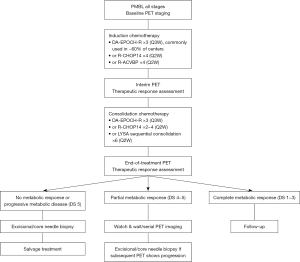
The place of CRT
Since several decades, CRT is widely used given the proven radiosensitivity of PMBL. The usefulness of CRT is currently highly debated, and several expert centers have abandoned it, although it is still widely used in many countries. However, several teams have expressed concern about the long-term toxicity of CRT, including the possible induction of secondary cancers, especially in a young population (<40 years old) of females (127-129). The doses, target volumes and methods of delivery of these mediastinal irradiations are very heterogeneous among centers and have not been the subject of randomized studies. Several groups still apply CRT for all patients after standard chemotherapy (114,130,131), whereas others restrict its use to cases in which there is partial response on PET at the end of treatment (103,132,133), and others have discontinued its use (134-136). Gleeson et al. reported in a subgroup analysis of UK NCRI that combining R-CHOP14 plus CRT offers a better outcome than R-CHOP21 plus CRT (5).
In the retrospective LYSA study, the number of patients who were assigned to CRT was very low (n=17) and became null after 2014, probably due to a change in the procedures used in LYSA centers (18). The landmark study reported by Dunleavy et al. 10 years ago established that treatment with intensified immunochemotherapy (DA-EPOCH-R) made the use of CRT unnecessary (4). Hayden et al. also observed in a homogeneous series of PMBL patients who received frontline R-CHOP that a PET-guided strategy may decrease the need for CRT in most patients (103). Of note, radiotherapy may play a relevant role in the treatment of patients who show localized mediastinal progression at the end of treatment. Indeed, Vassilakopoulos et al. reported sustainable control of the disease in most patients with PET positivity at the end of treatment when consolidated with radiotherapy, and a Deauville score of 5 or 4 with high uptake (SUVmax ≥5) after subsequent radiotherapy is predictive of failure (100). Patients with elevated FDG uptake (SUVmax ≥10) after R-CHOP might switch to CMR after mediastinal irradiation (100).
Several sets of data suggest that CRT may be abandoned in patients who are assigned to receive frontline dose-intense immunochemotherapy to avoid the long-term side effects of CRT (137-140). In addition, the common extra mediastinal relapses observed in the recent retrospective LYSA study do not offer support for the use of CRT (18). However, because real-life studies of large cohorts of PMBL treated with dose-dense chemotherapy with or without CRT are lacking, there is no unanimity regarding the exclusion of CRT. The results of the IELSG-37 randomized study (NCT01599559) may soon end the debate regarding CRT in PET negative (Deauville score 1–3) patients (141). However, this study will not provide any input regarding the use of CRT in end of treatment PET positive cases that are frequently false positives.
Management of relapsed/refractory PMBL
Relapsed/refractory patients represent a major unmet medical need. Contrary to the excellent prognosis observed in first-line treatment, the prognosis is very unfavorable in cases of disease progression, and progression occurs in approximately 10–20% of PMBL patients (18,142-144). Typically, progressions occur within the first year following the initiation of frontline treatment [primary refractory (PR) disease]; extramediastinal relapses can occur, but central nervous system or bone marrow involvement is uncommon. In cases of suspected disease progression, tumor biopsy is strongly recommended to exclude PET false positivity, to look for PDL1 overexpression by IHC (if not performed at initial diagnosis), to reassess CD19 and CD20 expression and to search for a composite or sequential tumoral contingent with MGZL or cHL features. Indeed, sequential PMBL and NS-cHL may occur, and some such cases have been demonstrated to be of common clonal origin and may have poorer outcomes (44-46,145,146). Salvage radiation therapy may be considered in nonbulky isolated mediastinal disease progression if patients do not receive CRT during their first-line treatment, but a low level of evidence for this approach exists (131). Table 4 outlines the effectiveness of the main R/R treatment options, and Figure 2 proposes a treatment algorithm for R/R PMBL patients.
Table 4
Reference | No. of patients | Study design | IPI | Salvage regimen | Response rate | EFS/PFS/TTP | OS |
---|---|---|---|---|---|---|---|
(147) | 27 | Phase II | aaIPI ≤1: 44%; aaIPI ≥2: 56% | Camrelizumab (anti-PD1) plus gemcitabine, vinorelbine, and pegylated liposomal doxorubicin | ORR: 74.1%; CR: 55.6% | 2-year PFS: 48.2% | 2-year OS: 81.5% |
(148) | KEYNOTE-013: n=21; KEYNOTE-170: n=53 | KEYNOTE-013: phase Ib; KEYNOTE-170: phase II | NA | Pembrolizumab single-agent | KEYNOTE-013: ORR: 48%, CR: 33%; KEYNOTE-170: ORR: 45%, CR: 13% | KEYNOTE-013: 1-year PFS: 47%; KEYNOTE-170: 1-year PFS: 38% | KEYNOTE-013: 1-year OS: 65%; KEYNOTE-170: 1-year OS: 58% |
(149) | 30 | CHECKMATE-436: phase I/II | NA | Nivolumab plus brentuximab-vedotin | ORR: 73%, CR: 37% | 6-month PFS: 63.5% | 6-month OS: 86.3% |
(150) | 28 | Retrospective | NA | Allo-SCT various conditioning regimens | NA | All patients: 2-year PFS: 39%; patients in CR or PR before allo-SCT: 2-year PFS: 50% | All patients: 2-year OS: 45%; patients in CR or PR before allo-SCT: 2-year OS: 58% |
(21) | 60 | Retrospective | IPI 0–1: 58%; IPI 2: 18%; IPI 3: 12%; IPI 4–5: 12% | R-ICE (48%); ICE (33%); O-DHAP (8%); ICEMAN (5%); other (12%); radiotherapy (87%); ASCT (85%) | ORR: 65%; CR: 40%; PR: 25% | All patients: 3-year EFS: 57%; transplanted patients: 3-year PFS: 60% | All patients: 3-year OS: 61%; transplanted patients: 3-year OS: 65% |
(125) | 86 | Retrospective | NA | BEAM-ASCT: 75%; other (27%); radiotherapy (31%) | CR: 58% | 3-year PFS: 62% | 3-year OS: 75% |
(151) | 33 | Retrospective | NA | Axicabtagene ciloleucel (100%); checkpoint blockade (57.6%) | ORR: 78%, CR: 69% | 2-year PFS: 64% | 2-year OS: 78% |
(143) | 37 | Retrospective | IPI 0–1: 50%; IPI 2–3: 44%; IPI 4–5: 6% | DHAP: 51%; ESHAP: 27%; GDP: 11%; Mini-BEAM: 5%; other (5%); proceed to ASCT: 22% | ORR: 25% | All patients: NA; post-ASCT: 2-year PFS: 57% | 2-year OS post progression: 15%; post-ASCT: 2-year OS: 67% |
PMBL, primary mediastinal B-cell lymphoma; aaIPI, age-adjusted international prognostic index; ORR, overall response rate; CR, complete response; EFS, event-free survival; PFS, progression-free survival; TTP, time to progression; OS, overall survival; NA, not available; allo-SCT, allogeneic stem cell transplantation; PR, partial response; R, rituximab; ICE, ifosfamide, carboplatin, etoposide; O-DHAP, ofatumumab, dexamethasone, high-dose Ara-C (cytarabine), cisplatin; ICEMAN, ifosfamide, carboplatin, etoposide, methotrexate, cytarabine; ASCT, autologous stem cell transplantation; BEAM, carmustine (BCNU), etoposide, cytarabine, melphalan; DHAP, dexamethasone, high-dose Ara-C (cytarabine), cisplatin; ESHAP, etoposide, methylprednisolone (solumedrol), high-dose cytarabine (ara-C) and cisplatin; GDP, gemcitabine, dexamethasone, cisplatin.
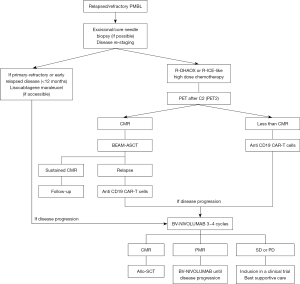
Treatment intensification through ASCT
The current standard approach to second-line treatment of “fit” patients is salvage high-dose platinum-based chemotherapy (R-DHAX, R-ICE or equivalent) followed by treatment intensification with ASCT (21,125). In the prerituximab era, outcomes in PR patients were very poor, with a 2-year survival probability of less than 10% (152). Then, in 1998, Sehn et al. described the outcomes of 35 PMBL patients with either frontline unfavorable prognostic characteristics or disease progression who were retreated with high-dose chemotherapy followed by ASCT [these patients received a carmustine, etoposide, and cyclophosphamide (CBV) conditioning regimen]. These high-risk PMBL patients had long-term disease-free survival (DFS) (5-year PFS: 83% in first-line patients vs. 58% in refractory patients vs. 27% in relapsed patients, P=0.02); therefore, this intensified approach became the standard of care for R/R-eligible patients (153). Of note, as in other lymphomas, the most relevant prognostic feature was chemosensitivity before transplant (risk ratio: 3.60; 95% CI: 1.14–11.4).
However, R/R PMBL is often resistant to second-line high-dose chemotherapy; therefore, patients will ultimately either not undergo ASCT because of salvage treatment failure or display early disease progression after ASCT. Indeed, Aoki et al. (144) reported a cohort of 44 PMBL patients diagnosed between 1996 and 2012 in which no frontline rituximab was administered to some patients and observed a 4-year PFS probability of 61% after second-line treatment intensification. In this study, chemorefractory patients (n=13) who did not respond to second-line high-dose chemotherapy had inferior outcomes compared to chemosensitive patients (n=31; 4-year OS: 80% vs. 50%, P=0.018). To add further data regarding the relevance of treatment intensification in R/R PMBL, we should mention the retrospective MD Anderson study that was reported in abstract form at American Society of Hematology (ASH) 2020; it included 58 R/R PMBL patients who received high-dose chemotherapy followed by an intensive ASCT conditioning regimen (rituximab/gemcitabine/busulfan/melphalan +/− vorinostat: R-GemBuMel) and CRT for patients with active disease at the time of transplant. This dose-intensified combination significantly improved event-free survival (EFS) and OS compared to the rituximab-BEAM conditioning regimen: two-thirds of the patients had long-term disease control, but these monocentric findings warrant further validation.
To conclude, therapeutic intensification with ASCT remains a potentially curative option for a subgroup of chemosensitive R/R PMBL patients if a CMR can be obtained before transplantation.
Immune checkpoint inhibitors
Anti-PD1 monotherapy
Nivolumab and pembrolizumab are the two main FDA-approved anti-PD1 checkpoint inhibitors used to treat hematological malignancies. Pembrolizumab is a humanized, highly selective, IgG4/kappa monoclonal antibody targeting PD-1. The common 9p24.1 rearrangements in PMBL karyotypes resulting in PDL1 and PDL2 overexpression by lymphoma cells support the use of anti-PD-1 blockers in this disease.
In the pivotal phase II KEYNOTE-170 study, pembrolizumab was tested in a population of R/R PMBL patients who had already received 2 prior treatments and then relapsed or were unfit for ASCT; these patients were designated as having the highest unmet medical need. The patients were assigned to receive a pembrolizumab infusion of 200 mg every 3 weeks for a maximum of 35 cycles or for up to 2 years until proven disease progression or limiting toxicity occurred. The overall response rate was estimated to be 45% (95% CI: 32–60%): 7 and 10 patients reached CR as assessed using the Cheson 2007 criteria (13%) or the Lugano 2014 criteria (19%), respectively (148,154). The median time until a response to pembrolizumab was observed was 2.9 months (range, 2.4–8.5 months). After a median follow-up of three and a half years, the median PFS and OS were 5.5 and 22.3 months, respectively, with 3-year PFS and OS probabilities of 36% and 45%, respectively. The median duration of response was not achieved (range, 1.1–46.9 months), and three-quarters of the patients displayed a durable response that lasted longer than 36 months. Interestingly, the therapeutic response was correlated with overexpression of PDL1 as evaluated using IHC (such overexpression is itself related to the presence of 9p24.1 rearrangements in cytogenetics), suggesting that IHC could be a predictive marker of the response in these patients. The safety profile of the treatment regimen appeared excellent, as the most frequent adverse event was neutropenia (13%). Severe immune-related adverse event (AE) occurred in only 2 patients (grade 3 myositis: n=1; grade 4 pneumonitis: n=1) and did not result in definitive treatment interruption. Given the positive benefit/risk profile of pembrolizumab observed in this phase II study, the FDA approved this molecule in 2018 for use in R/R PMBL patients after two or more previous lines of treatment. However, no EMA approval was obtained, probably due to the lack of a comparator arm in the phase II study, and this treatment has been approved only in Europe for R/R cHL patients. Camrelizumab, another anti PD1 checkpoint inhibitor, also demonstrated an interesting efficacy profile in combination with salvage chemotherapy (gemcitabine, vinorelbine, pegylated liposomal doxorubicin) with 2-year PFS and OS probabilities of 48.2% and 81.5%, respectively (147). This drug was recently approved in China for the treatment of R/R cHL and is currently developed in B-cell lymphoma and other malignancies.
Nivolumab plus brentuximab vedotin
The use of a combination of the anti-CD30 antibody-drug conjugate BV and nivolumab was first assessed in patients with cHL, in whom CD30 positivity is a common feature. In a pivotal trial, this association showed 82% overall response rate (ORR) and 61% CR, with an acceptable safety profile, potentially providing patients with R/R cHL an alternative to traditional chemotherapy (155). However, this combination is still unapproved by the FDA for R/R cHL, likely because an improvement in patient-reported outcomes or survival has not yet been established. CD30 staining in PMBL is lower and more heterogeneous than in cHL. In the Checkmate 436 trial, heavily pretreated R/R PMBL patients received BV at 1.8 mg/kg and a fixed dose of 240 mg nivolumab every 3 weeks until disease progression or unacceptable toxicity occurred. ASCT was performed at the discretion of the investigators. The ORR was 73%, and the CR rate was 37%. The times to first objective response and CR were remarkably short (median 1.3 and 3.2 months, respectively), and the median duration of CR was unattained. Eleven patients who responded to this combination then underwent treatment intensification (six allogeneic stem cell transplants, five ASCT). Nivolumab plus BV was well tolerated, with the most frequent adverse events consisting of severe neutropenia (grade 3–4: 30%) and peripheral neuropathy (overall: 27%; grade 3–4: 10%, three patients discontinued BV). Two patients experienced immune-related adverse events: one had grade 3 colitis and rash, and one had grade 4 hepatitis (149). Zinzani et al. reported a very low objective response rate of BV as a single agent in a phase II trial (13% in R/R PMBL) (156). Therefore, a biological synergistic effect likely explains the efficacy of nivolumab plus BV; the effect probably occurs through depletion of T-reg lymphocytes and initiation of immunogenic cell death by BV, amplified by PD-1 blockade (157,158). Despite an overall favorable risk/benefit profile, the combination of nivolumab and BV remains unapproved by the FDA and the EMA for R/R PMBL, likely due to the lack of comparative data and mature survival data. However, this combination is frequently used in many lymphoma centers due to the chemorefractoriness of R/R PMBL. This combination should also be further explored as a bridge to CAR-T-cell therapy, as reported in an abstract by Chiappella et al. at ASH 2021. In this real-life report of CAR-T-cell use in R/R PMBL patients, 18 patients underwent apheresis, including 3 patients exposed to nivolumab plus BV, and no manufacturing failure was observed. Of note, the authors observed no difference in cytokine release syndrome or neurotoxicity between patients who had previously been exposed to checkpoint inhibitors and those who had not (159).
CAR-T cells
Since 2018, three anti-CD19 CAR-T cell therapies have been commercialized for R/R DLBCL patients who have received two prior lines of treatment. Axi-cel is approved for use in R/R PMBL patients based on the results of the ZUMA-1 trial, but only eight patients with R/R PMBL were enrolled in this trial (160). Of note, tisa-cel is not officially approved for use in PMBL patients because the relevant histology was not included in the pivotal JULIET trial (161). In the TRANSCEND-NHL-001 trial, 14 R/R PMBL patients received lisocabtagene maraleucel (liso-cel) and subsequently showed ORR and CRR of 79% and 50%, respectively (162). Available data regarding the real-life efficacy of treatment with CAR-T cells in the R/R PMBL population are scarce. Of 46 patients treated with axi-cel between 2009 and 2015 in America, six had PMBL, and only 5 were evaluable for response, including two who had CR and in whom the durations of response were longer than 97 and 38 months, two who had stable disease and one who had progressive disease (ORR: 40%) (163). More recently, Crombie et al. reported the real-world outcomes of 33 R/R PMBL patients in five American centers who received axi-cel between 2018 and 2019 (151). The patients in this cohort received a median of 3 (range, 1–9) prior lines of therapy; 30% had received ASCT, and 67% had received radiotherapy. Among the 32 patients evaluable for response, ORR was 78% and CR was 69%, leading to 2-year PFS and OS rates of 64% and 78%, respectively. Interestingly, nineteen patients also received anti-PD1 therapy either before (n=14), after (n=4), or both before and after (n=1) axi-cel infusion. The ORR, CR, PFS, OS, cytokine release syndrome (CRS) and neurotoxicity rates were similar in patients with different sequences of anti-PD1 exposure. One patient with progressive disease following anti-PD1 and axi-cel therapy achieved a CR when retreated with anti-PD1 in the post-CAR-T cell setting, but the authors admitted the inadequacy of this single observation in assessing the benefit of administering anti-PD1 treatment after axi-cel therapy (151).
Finally, the management of refractory PMBL is changing in light of the recent FDA-approval of CAR-T cell (liso-cel) for LBCL in the second line. The ZUMA-7 study has recently established the superiority of axi-cel vs. standard of care (high-dose chemotherapy and ASCT) in refractory/early relapsed LBL but excluding PMBL. Meanwhile, the TRANSFORM study compared liso-cel vs. standard of care in several LBL subtypes and included PMBL patients. This phase III trial demonstrated that median EFS was significantly improved with liso-cel [10.1 months (95% CI: 6.1–not reached)] compared with the standard of care [2.3 months (95% CI: 2.2–4.3)]; stratified hazard ratio 0·35; 95% CI: 0.23–0.53; P<0.0001). These results led to the approval of liso-cel for PMBL patients who have refractory disease to first-line chemoimmunotherapy or relapse within 12 months of first-line chemoimmunotherapy.
Allogeneic stem cell transplantation
Because PMBL is an uncommon disease, most of the data that support allogeneic stem cell transplantation have been extrapolated from DLBCL studies, particularly studies of patients who relapsed after ASCT (164-167). However, two recent studies support the utility of allogeneic stem cell transplantation in R/R PMBL. First, Herrera et al. reported 28 patients in 3 American centers who presented encouraging results of 45% 5-year OS and 34% 5-year DFS probabilities (150). More recently, Le Calvez et al., on behalf of the Société Francophone de Greffe de Moelle et de Thérapie Cellulaire (SFGM-TC) and the LYSA group, reported in an abstract the 2-year outcomes of 33 R/R PMBL patients. OS, PFS, nonrelapse mortality, graft-versus-host DFS/RFS and cumulative incidence of relapse at 2 years were 48% (95% CI: 33–70%), 47% (95% CI: 33–68%), 18% (95% CI: 7–34%), 38.5% (95% CI: 25–60%), and 34% (95% CI: 18–50%), respectively (168). The two series displayed similar encouraging results, and the observed plateaus of the survival curves suggested long-term disease control. To conclude, allogeneic stem cell transplantation is a valuable therapeutic option in a selected subset of treatment-sensitive R/R patients who experience disease progression after ASCT. Further studies are warranted to assess its clinical relevance and the appropriate timing of its use in the CAR-T-cell/immunotherapy era.
To conclude, the landscape of R/R PMBL is currently changing with the approval of CAR-T cells in second line in refractory disease and the impressive results of checkpoint blockades. The order and better use sequence of checkpoint inhibitors and CAR-T cells therapies remains strongly debated, as some patients can have sustained remissions with checkpoint inhibitors, with no need for additional treatments.
Circulating tumor DNA (ctDNA) in PMBL
The role of ctDNA as a reliable biomarker of minimal residual disease (MRD) in cHL was recently established in several studies (14,169,170). In particular, using phased variant-enhanced CAPP-seq high-throughput sequencing technology, Pirosa et al. demonstrated that MRD combined with interim PET had significantly superior accuracy for the anticipation of progression or relapse compared to MRD or interim PET alone in cHL patients (170).
Earlier primary chemoresistance detection and predictive biomarker identification would also be very relevant in the PMBL population to (I) improve outcomes of R/R patients; (II) avoid useless second-line chemotherapy in the subset of refractory patients; and (III) promote the use of effective alternatives such as immunotherapy. Given that interim and end-of-treatment PET is often difficult to interpret in this disease, we may hypothesize that ctDNA measurement would help refine PET-assessed therapeutic response and would allow more personalized care based on minimal residual disease (MRD). With the past 5 years, several groups have established that ctDNA can be quite easily measured in blood samples from DLBCL, cHL and PMBL patients using high-throughput sequencing methods (14,171,172). More recently, Pang et al. monitored ctDNA in 16 PMBL patients receiving frontline chemotherapy and demonstrated that 94% of the patients displayed full clearance of plasma ctDNA after 2 cycles of chemotherapy and that 3 of 4 relapsing cases had measurable ctDNA 2 weeks before the detection of disease progression on follow-up imaging (173). The ongoing CAMIL (NCT04824950) study is dedicated to assessing the positive and negative predictive values of ctDNA versus PET to anticipate PR disease after 2 or 4 cycles of frontline chemotherapy. The results of this study will help provide a solid background for anticipated salvage immunotherapy (anti-PD1 checkpoint inhibition) in early MRD-positive high-risk PMBL patients. As PMBL is highly curable, it is legitimate to consider therapeutic de-escalation strategies and to consider limiting the use of ASCT to late metabolic responders (who are at higher risk of relapse), but the availability of a biomarker that is complementary to PET would be useful in the stratification of patients. Liquid biopsy or monitoring of ctDNA could be used to better assess the therapeutic response, allowing reduction of ASCT if ctDNA is undetectable after 2 and 4 cycles of chemotherapy. In addition, identifying early PR disease that occurs in 10% of patients with very poor OS (<50% at 2 years) is crucial. The use of ctDNA would allow the identification of PR patients after only 2 cycles of chemotherapy and would support timely recommendation for salvage immunotherapy. Several studies of ctDNA-based MRD in DLBCL patients have been reported, but there is no study dedicated to PMBL, although there are particular issues in the management of these patients, especially the interpretation of mediastinal uptake on PET. In the literature, the proportion of false-positive lesions based on interim and end-of-treatment PET images ranges from 11% (174) to 20% (20). A multicentric prospective study (NCT04824950) designed to evaluate the relevance of MRD monitoring in addition to PET in PMBL is ongoing. The results of this study may provide a solid rationale for the use of ctDNA monitoring in PMBL patients. The study may also provide a foundation for a future interventional trial based on the use of real-time MRD monitoring to de-escalate treatment intensity in early MRD-negative patients or to reorient treatment strategies toward immunotherapy in cases of early molecular progression.
Future directions
To date, the main studies of the genetic landscape and phenotype of PMBL cells have reported a high prevalence of 9p24 rearrangements leading to PDL1 and PDL2 protein overexpression. As in cHL, this recurrent abnormality is favorable to the introduction of checkpoint inhibitors in R/R PMBL. However, more detailed dissection of the tumor microenvironment of PMBL remains a major need. Indeed, immune cell infiltration of PMBL tumors has been analyzed on a small scale using IHC, and the analysis indicates lower T-cell infiltration and a rather strong CD163+ mononuclear-phagocyte compartment compared to cHL (175). Although the T-cell compartment of cHL tumors has been broadly described using mass cytometry (176), such studies are still lacking in PMBL, probably due to the paucity of the biopsy material remaining after histopathological diagnosis of small samples, an issue related to the mediastinal location of PMBL.
Regarding frontline treatment, we can anticipate that the medical community will move toward a progressive deintensification of first-line strategies, reducing the use of ASCT and CRT and adopting an integrated risk-adapted and response-adapted treatment program. We also hypothesize that in the next 5 years immunotherapy with anti-PD1/PDL-1 will be introduced as a first-line treatment in combination with a reduced number of chemotherapy cycles. We should also reconsider the use of CRT after immunochemotherapy in the large majority of patients, especially in patients with bulky mediastinal masses and residual mediastinal uptake at the end of treatment. A more personalized approach that includes MRD monitoring should progressively be integrated to include assessment of the quality and quantification of the molecular response in the decision-making regarding consolidation treatments.
Conclusions
PMBL is a specific entity of LBCL with clinical and biological characteristics close to those of cHL, and treatment of individuals with PMBL is similar to that of young DLBCL patients. PMBL has a particular natural history and a singular biology that make it unique. Its low incidence is responsible for its exclusion from many therapeutic CTs (most of which primarily include DLBCL patients) and the absence of a consensus regarding the management of these lymphomas. However, PMBL occurs in a population of young patients with specific concerns, and we should continue our efforts to develop new and innovative therapeutic strategies for treatment of these lymphomas, particularly with the use of MRD-guided treatments and immunotherapies such as anti-PD1 and CAR-T cells.
Acknowledgments
Funding: None.
Footnote
Conflicts of Interest: All authors have completed the ICMJE uniform disclosure form (available at https://aol.amegroups.com/article/view/10.21037/aol-22-13/coif). VC serves as an unpaid editorial board member of Annals of Lymphoma from March 2022 to February 2024. The other authors have no conflicts of interest to declare.
Ethical Statement: The authors are accountable for all aspects of the work in ensuring that questions related to the accuracy or integrity of any part of the work are appropriately investigated and resolved.
Open Access Statement: This is an Open Access article distributed in accordance with the Creative Commons Attribution-NonCommercial-NoDerivs 4.0 International License (CC BY-NC-ND 4.0), which permits the non-commercial replication and distribution of the article with the strict proviso that no changes or edits are made and the original work is properly cited (including links to both the formal publication through the relevant DOI and the license). See: https://creativecommons.org/licenses/by-nc-nd/4.0/.
References
- Swerdlow SH, Campo E, Pileri SA, et al. The 2016 revision of the World Health Organization classification of lymphoid neoplasms. Blood 2016;127:2375-90. [Crossref] [PubMed]
- Liu PP, Wang KF, Xia Y, et al. Racial patterns of patients with primary mediastinal large B-cell lymphoma: SEER analysis. Medicine (Baltimore) 2016;95:e4054. [Crossref] [PubMed]
- Massoud M, Koscielny S, Lapusan S, et al. Primary mediastinal large B-cell lymphomas treated with dose-intensified CHOP alone or CHOP combined with radiotherapy. Leuk Lymphoma 2008;49:1510-5. [Crossref] [PubMed]
- Dunleavy K, Pittaluga S, Maeda LS, et al. Dose-adjusted EPOCH-rituximab therapy in primary mediastinal B-cell lymphoma. N Engl J Med 2013;368:1408-16. [Crossref] [PubMed]
- Gleeson M, Hawkes EA, Cunningham D, et al. R-CHOP in Primary Mediastinal B-Cell Lymphoma (PMBL): Results from the UK NCRI R-CHOP 14 v 21 Trial. Blood 2015;126:2689. [Crossref]
- Rosenwald A, Wright G, Leroy K, et al. Molecular diagnosis of primary mediastinal B cell lymphoma identifies a clinically favorable subgroup of diffuse large B cell lymphoma related to Hodgkin lymphoma. J Exp Med 2003;198:851-62. [Crossref] [PubMed]
- Green MR, Monti S, Rodig SJ, et al. Integrative analysis reveals selective 9p24.1 amplification, increased PD-1 ligand expression, and further induction via JAK2 in nodular sclerosing Hodgkin lymphoma and primary mediastinal large B-cell lymphoma. Blood 2010;116:3268-77. [Crossref] [PubMed]
- Twa DD, Steidl C. Structural genomic alterations in primary mediastinal large B-cell lymphoma. Leuk Lymphoma 2015;56:2239-50. [Crossref] [PubMed]
- Roemer MG, Advani RH, Ligon AH, et al. PD-L1 and PD-L2 Genetic Alterations Define Classical Hodgkin Lymphoma and Predict Outcome. J Clin Oncol 2016;34:2690-7. [Crossref] [PubMed]
- Twa DD, Chan FC, Ben-Neriah S, et al. Genomic rearrangements involving programmed death ligands are recurrent in primary mediastinal large B-cell lymphoma. Blood 2014;123:2062-5. [Crossref] [PubMed]
- Mottok A, Hung SS, Chavez EA, et al. Integrative genomic analysis identifies key pathogenic mechanisms in primary mediastinal large B-cell lymphoma. Blood 2019;134:802-13. [Crossref] [PubMed]
- Chapuy B, Stewart C, Dunford AJ, et al. Genomic analyses of PMBL reveal new drivers and mechanisms of sensitivity to PD-1 blockade. Blood 2019;134:2369-82. [Crossref] [PubMed]
- Tiacci E, Döring C, Brune V, et al. Analyzing primary Hodgkin and Reed-Sternberg cells to capture the molecular and cellular pathogenesis of classical Hodgkin lymphoma. Blood 2012;120:4609-20. [Crossref] [PubMed]
- Spina V, Bruscaggin A, Cuccaro A, et al. Circulating tumor DNA reveals genetics, clonal evolution, and residual disease in classical Hodgkin lymphoma. Blood 2018;131:2413-25. [Crossref] [PubMed]
- Reichel J, Chadburn A, Rubinstein PG, et al. Flow sorting and exome sequencing reveal the oncogenome of primary Hodgkin and Reed-Sternberg cells. Blood 2015;125:1061-72. [Crossref] [PubMed]
- Aoki T, Izutsu K, Suzuki R, et al. Prognostic significance of pleural or pericardial effusion and the implication of optimal treatment in primary mediastinal large B-cell lymphoma: a multicenter retrospective study in Japan. Haematologica 2014;99:1817-25. [Crossref] [PubMed]
- Dunleavy K. Primary mediastinal B-cell lymphoma: biology and evolving therapeutic strategies. Hematology Am Soc Hematol Educ Program 2017;2017:298-303. [Crossref] [PubMed]
- Camus V, Rossi C, Sesques P, et al. Outcomes after first-line immunochemotherapy for primary mediastinal B-cell lymphoma: a LYSA study. Blood Adv 2021;5:3862-72. [Crossref] [PubMed]
- Adams HJA, Kwee TC. Proportion of false-positive lesions at interim and end-of-treatment FDG-PET in lymphoma as determined by histology: Systematic review and meta-analysis. Eur J Radiol 2016;85:1963-70. [Crossref] [PubMed]
- Melani C, Advani R, Roschewski M, et al. End-of-treatment and serial PET imaging in primary mediastinal B-cell lymphoma following dose-adjusted EPOCH-R: a paradigm shift in clinical decision making. Haematologica 2018;103:1337-44. [Crossref] [PubMed]
- Vardhana S, Hamlin PA, Yang J, et al. Outcomes of Relapsed and Refractory Primary Mediastinal (Thymic) Large B Cell Lymphoma Treated with Second-Line Therapy and Intent to Transplant. Biol Blood Marrow Transplant 2018;24:2133-8. [Crossref] [PubMed]
- Zinzani PL, Martelli M, Poletti V, et al. Practice guidelines for the management of extranodal non-Hodgkin's lymphomas of adult non-immunodeficient patients. Part I: primary lung and mediastinal lymphomas. A project of the Italian Society of Hematology, the Italian Society of Experimental Hematology and the Italian Group for Bone Marrow Transplantation. Haematologica 2008;93:1364-71. [Crossref] [PubMed]
- Cazals-Hatem D, Lepage E, Brice P, et al. Primary mediastinal large B-cell lymphoma. A clinicopathologic study of 141 cases compared with 916 nonmediastinal large B-cell lymphomas, a GELA ("Groupe d'Etude des Lymphomes de l'Adulte") study. Am J Surg Pathol 1996;20:877-88. [Crossref] [PubMed]
- Wilson WH, Pittaluga S, Nicolae A, et al. A prospective study of mediastinal gray-zone lymphoma. Blood 2014;124:1563-9. [Crossref] [PubMed]
- Lekovic D, Miljic P, Mihaljevic B. Increased risk of venous thromboembolism in patients with primary mediastinal large B-cell lymphoma. Thromb Res 2010;126:477-80. [Crossref] [PubMed]
- Zimmerman S, Davis M. Rapid Fire: Superior Vena Cava Syndrome. Emerg Med Clin North Am 2018;36:577-84. [Crossref] [PubMed]
- Patriarcheas V, Grammoustianou M, Ptohis N, et al. Malignant Superior Vena Cava Syndrome: State of the Art. Cureus 2022;14:e20924. [Crossref] [PubMed]
- Ratzon R, Tamir S, Friehmann T, et al. Thrombosis, anticoagulation and outcomes in malignant superior vena cava syndrome. J Thromb Thrombolysis 2019;47:121-8. [Crossref] [PubMed]
- Farge D, Frere C, Connors JM, et al. 2022 international clinical practice guidelines for the treatment and prophylaxis of venous thromboembolism in patients with cancer, including patients with COVID-19. Lancet Oncol 2022;23:e334-47. [Crossref] [PubMed]
- Paulli M, Sträter J, Gianelli U, et al. Mediastinal B-cell lymphoma: a study of its histomorphologic spectrum based on 109 cases. Hum Pathol 1999;30:178-87. [Crossref] [PubMed]
- Kanavaros P, Gaulard P, Charlotte F, et al. Discordant expression of immunoglobulin and its associated molecule mb-1/CD79a is frequently found in mediastinal large B cell lymphomas. Am J Pathol 1995;146:735-41. [PubMed]
- Pileri SA, Gaidano G, Zinzani PL, et al. Primary mediastinal B-cell lymphoma: high frequency of BCL-6 mutations and consistent expression of the transcription factors OCT-2, BOB.1, and PU.1 in the absence of immunoglobulins. Am J Pathol 2003;162:243-53. [Crossref] [PubMed]
- al-Sharabati M, Chittal S, Duga-Neulat I, et al. Primary anterior mediastinal B-cell lymphoma. A clinicopathologic and immunohistochemical study of 16 cases. Cancer 1991;67:2579-87. [Crossref] [PubMed]
- Davis RE, Dorfman RF, Warnke RA. Primary large-cell lymphoma of the thymus: a diffuse B-cell neoplasm presenting as primary mediastinal lymphoma. Hum Pathol 1990;21:1262-8. [Crossref] [PubMed]
- Brandter LB, Smith CI, Hammarström L, et al. Clonal immunoglobulin gene rearrangements in primary mediastinal clear cell lymphomas. Leukemia 1989;3:122-9. [PubMed]
- Möller P, Moldenhauer G, Momburg F, et al. Mediastinal lymphoma of clear cell type is a tumor corresponding to terminal steps of B cell differentiation. Blood 1987;69:1087-95. [Crossref] [PubMed]
- Calaminici M, Piper K, Lee AM, et al. CD23 expression in mediastinal large B-cell lymphomas. Histopathology 2004;45:619-24. [Crossref] [PubMed]
- Salama ME, Rajan Mariappan M, Inamdar K, et al. The value of CD23 expression as an additional marker in distinguishing mediastinal (thymic) large B-cell lymphoma from Hodgkin lymphoma. Int J Surg Pathol 2010;18:121-8. [Crossref] [PubMed]
- Panjwani PK, Charu V, DeLisser M, et al. Programmed death-1 ligands PD-L1 and PD-L2 show distinctive and restricted patterns of expression in lymphoma subtypes. Hum Pathol 2018;71:91-9. [Crossref] [PubMed]
- Pileri SA, Zinzani PL, Gaidano G, et al. Pathobiology of primary mediastinal B-cell lymphoma. Leuk Lymphoma 2003;44:S21-6. [Crossref] [PubMed]
- Bledsoe JR, Redd RA, Hasserjian RP, et al. The immunophenotypic spectrum of primary mediastinal large B-cell lymphoma reveals prognostic biomarkers associated with outcome. Am J Hematol 2016;91:E436-41. [Crossref] [PubMed]
- Copie-Bergman C, Gaulard P, Maouche-Chrétien L, et al. The MAL gene is expressed in primary mediastinal large B-cell lymphoma. Blood 1999;94:3567-75. [Crossref] [PubMed]
- Copie-Bergman C, Plonquet A, Alonso MA, et al. MAL expression in lymphoid cells: further evidence for MAL as a distinct molecular marker of primary mediastinal large B-cell lymphomas. Mod Pathol 2002;15:1172-80. [Crossref] [PubMed]
- Campo E, Jaffe ES, Cook JR, et al. The International Consensus Classification of Mature Lymphoid Neoplasms: a report from the Clinical Advisory Committee. Blood 2022;140:1229-53. [Crossref] [PubMed]
- Pittaluga S, Nicolae A, Wright GW, et al. Gene Expression Profiling of Mediastinal Gray Zone Lymphoma and Its Relationship to Primary Mediastinal B-cell Lymphoma and Classical Hodgkin Lymphoma. Blood Cancer Discov 2020;1:155-61. [Crossref] [PubMed]
- Aussedat G, Traverse-Glehen A, Stamatoullas A, et al. Composite and sequential lymphoma between classical Hodgkin lymphoma and primary mediastinal lymphoma/diffuse large B-cell lymphoma, a clinico-pathological series of 25 cases. Br J Haematol 2020;189:244-56. [Crossref] [PubMed]
- Yuan J, Wright G, Rosenwald A, et al. Identification of Primary Mediastinal Large B-cell Lymphoma at Nonmediastinal Sites by Gene Expression Profiling. Am J Surg Pathol 2015;39:1322-30. [Crossref] [PubMed]
- Bobée V, Ruminy P, Marchand V, et al. Determination of Molecular Subtypes of Diffuse Large B-Cell Lymphoma Using a Reverse Transcriptase Multiplex Ligation-Dependent Probe Amplification Classifier: A CALYM Study. J Mol Diagn 2017;19:892-904. [Crossref] [PubMed]
- Bobée V, Drieux F, Marchand V, et al. Combining gene expression profiling and machine learning to diagnose B-cell non-Hodgkin lymphoma. Blood Cancer J 2020;10:59. [Crossref] [PubMed]
- Aisenberg AC. Primary large cell lymphoma of the mediastinum. Semin Oncol 1999;26:251-8. [PubMed]
- Fend F, Nachbaur D, Oberwasserlechner F, et al. Phenotype and topography of human thymic B cells. An immunohistologic study. Virchows Arch B Cell Pathol Incl Mol Pathol 1991;60:381-8. [Crossref] [PubMed]
- Takaba H, Takayanagi H. The Mechanisms of T Cell Selection in the Thymus. Trends Immunol 2017;38:805-16. [Crossref] [PubMed]
- Gies V, Guffroy A, Danion F, et al. B cells differentiate in human thymus and express AIRE. J Allergy Clin Immunol 2017;139:1049-52.e12. [Crossref] [PubMed]
- Isaacson PG, Norton AJ, Addis BJ. The human thymus contains a novel population of B lymphocytes. Lancet 1987;2:1488-91. [Crossref] [PubMed]
- Nango K, Inaba M, Inaba K, et al. Ontogeny of thymic B cells in normal mice. Cell Immunol 1991;133:109-15. [Crossref] [PubMed]
- Montecino-Rodriguez E, Johnson A, Dorshkind K. Thymic stromal cells can support B cell differentiation from intrathymic precursors. J Immunol 1996;156:963-7. [Crossref] [PubMed]
- Akashi K, Richie LI, Miyamoto T, et al. B lymphopoiesis in the thymus. J Immunol 2000;164:5221-6. [Crossref] [PubMed]
- Perera J, Meng L, Meng F, et al. Autoreactive thymic B cells are efficient antigen-presenting cells of cognate self-antigens for T cell negative selection. Proc Natl Acad Sci U S A 2013;110:17011-6. [Crossref] [PubMed]
- Than S, Inaba M, Inaba K, et al. Origin of thymic and peritoneal Ly-1 B cells. Eur J Immunol 1992;22:1299-303. [Crossref] [PubMed]
- Yamano T, Nedjic J, Hinterberger M, et al. Thymic B Cells Are Licensed to Present Self Antigens for Central T Cell Tolerance Induction. Immunity 2015;42:1048-61. [Crossref] [PubMed]
- Perera J, Huang H. The development and function of thymic B cells. Cell Mol Life Sci 2015;72:2657-63. [Crossref] [PubMed]
- de Leval L, Ferry JA, Falini B, et al. Expression of bcl-6 and CD10 in primary mediastinal large B-cell lymphoma: evidence for derivation from germinal center B cells? Am J Surg Pathol 2001;25:1277-82. [Crossref] [PubMed]
- Tsang P, Cesarman E, Chadburn A, et al. Molecular characterization of primary mediastinal B cell lymphoma. Am J Pathol 1996;148:2017-25. [PubMed]
- Malpeli G, Barbi S, Moore PS, et al. Primary mediastinal B-cell lymphoma: hypermutation of the BCL6 gene targets motifs different from those in diffuse large B-cell and follicular lymphomas. Haematologica 2004;89:1091-9. [PubMed]
- Gunawardana J, Chan FC, Telenius A, et al. Recurrent somatic mutations of PTPN1 in primary mediastinal B cell lymphoma and Hodgkin lymphoma. Nat Genet 2014;46:329-35. [Crossref] [PubMed]
- Viganò E, Gunawardana J, Mottok A, et al. Somatic IL4R mutations in primary mediastinal large B-cell lymphoma lead to constitutive JAK-STAT signaling activation. Blood 2018;131:2036-46. [Crossref] [PubMed]
- Noerenberg D, Briest F, Hennch C, et al. S101: Genetic and epigenetic factors driving primary mediastinal B-cell lymphoma pathogenesis and outcome. HemaSphere 2022;6:2-3. [Crossref]
- Melzner I, Bucur AJ, Brüderlein S, et al. Biallelic mutation of SOCS-1 impairs JAK2 degradation and sustains phospho-JAK2 action in the MedB-1 mediastinal lymphoma line. Blood 2005;105:2535-42. [Crossref] [PubMed]
- Dubois S, Viailly PJ, Mareschal S, et al. Next-Generation Sequencing in Diffuse Large B-Cell Lymphoma Highlights Molecular Divergence and Therapeutic Opportunities: a LYSA Study. Clin Cancer Res 2016;22:2919-28. [Crossref] [PubMed]
- Ritz O, Guiter C, Castellano F, et al. Recurrent mutations of the STAT6 DNA binding domain in primary mediastinal B-cell lymphoma. Blood 2009;114:1236-42. [Crossref] [PubMed]
- Mansouri L, Noerenberg D, Young E, et al. Frequent NFKBIE deletions are associated with poor outcome in primary mediastinal B-cell lymphoma. Blood 2016;128:2666-70. [Crossref] [PubMed]
- Jardin F, Pujals A, Pelletier L, et al. Recurrent mutations of the exportin 1 gene (XPO1) and their impact on selective inhibitor of nuclear export compounds sensitivity in primary mediastinal B-cell lymphoma. Am J Hematol 2016;91:923-30. [Crossref] [PubMed]
- Leithäuser F, Bäuerle M, Huynh MQ, et al. Isotype-switched immunoglobulin genes with a high load of somatic hypermutation and lack of ongoing mutational activity are prevalent in mediastinal B-cell lymphoma. Blood 2001;98:2762-70. [Crossref] [PubMed]
- Popov SW, Moldenhauer G, Wotschke B, et al. Target sequence accessibility limits activation-induced cytidine deaminase activity in primary mediastinal B-cell lymphoma. Cancer Res 2007;67:6555-64. [Crossref] [PubMed]
- Stein H, Marafioti T, Foss HD, et al. Down-regulation of BOB.1/OBF.1 and Oct2 in classical Hodgkin disease but not in lymphocyte predominant Hodgkin disease correlates with immunoglobulin transcription. Blood 2001;97:496-501. [Crossref] [PubMed]
- Bentz M, Barth TF, Brüderlein S, et al. Gain of chromosome arm 9p is characteristic of primary mediastinal B-cell lymphoma (MBL): comprehensive molecular cytogenetic analysis and presentation of a novel MBL cell line. Genes Chromosomes Cancer 2001;30:393-401. [Crossref] [PubMed]
- Savage KJ, Monti S, Kutok JL, et al. The molecular signature of mediastinal large B-cell lymphoma differs from that of other diffuse large B-cell lymphomas and shares features with classical Hodgkin lymphoma. Blood 2003;102:3871-9. [Crossref] [PubMed]
- Möller P, Brüderlein S, Sträter J, et al. MedB-1, a human tumor cell line derived from a primary mediastinal large B-cell lymphoma. Int J Cancer 2001;92:348-53. [Crossref] [PubMed]
- Sambade C, Berglund M, Lagercrantz S, et al. U-2940, a human B-cell line derived from a diffuse large cell lymphoma sequential to Hodgkin lymphoma. Int J Cancer 2006;118:555-63. [Crossref] [PubMed]
- Nacheva E, Dyer MJS, Metivier C, et al. B-cell non-Hodgkin’s lymphoma cell line (Karpas 1106) with complex translocation involving 18q21.3 but lacking BCL2 rearrangement and expression. Blood 1994;84:3422-8. [Crossref] [PubMed]
- Tanaka Y, Maeshima AM, Nomoto J, et al. Expression pattern of PD-L1 and PD-L2 in classical Hodgkin lymphoma, primary mediastinal large B-cell lymphoma, and gray zone lymphoma. Eur J Haematol 2018;100:511-7. [Crossref] [PubMed]
- Guiter C, Dusanter-Fourt I, Copie-Bergman C, et al. Constitutive STAT6 activation in primary mediastinal large B-cell lymphoma. Blood 2004;104:543-9. [Crossref] [PubMed]
- Feuerhake F, Kutok JL, Monti S, et al. NFκB activity, function, and target-gene signatures in primary mediastinal large B-cell lymphoma and diffuse large B-cell lymphoma subtypes. Blood 2005;106:1392-9. [Crossref] [PubMed]
- Steidl C, Shah SP, Woolcock BW, et al. MHC class II transactivator CIITA is a recurrent gene fusion partner in lymphoid cancers. Nature 2011;471:377-81. [Crossref] [PubMed]
- Mottok A, Woolcock B, Chan FC, et al. Genomic Alterations in CIITA Are Frequent in Primary Mediastinal Large B Cell Lymphoma and Are Associated with Diminished MHC Class II Expression. Cell Rep 2015;13:1418-31. [Crossref] [PubMed]
- Roemer MG, Advani RH, Redd RA, et al. Classical Hodgkin Lymphoma with Reduced β2M/MHC Class I Expression Is Associated with Inferior Outcome Independent of 9p24.1 Status. Cancer Immunol Res 2016;4:910-6. [Crossref] [PubMed]
- Tuveri S, Debackere K, Marcelis L, et al. Primary mediastinal large B-cell lymphoma is characterized by large-scale copy-neutral loss of heterozygosity. Genes Chromosomes Cancer 2022;61:603-15. [Crossref] [PubMed]
- Duns G, Viganò E, Ennishi D, et al. Characterization of DLBCL with a PMBL gene expression signature. Blood 2021;138:136-48. [Crossref] [PubMed]
- Tilly H, Gomes da Silva M, Vitolo U, et al. Diffuse large B-cell lymphoma (DLBCL): ESMO Clinical Practice Guidelines for diagnosis, treatment and follow-up. Ann Oncol 2015;26:v116-25. [Crossref] [PubMed]
- Vitolo U, Seymour JF, Martelli M, et al. Extranodal diffuse large B-cell lymphoma (DLBCL) and primary mediastinal B-cell lymphoma: ESMO Clinical Practice Guidelines for diagnosis, treatment and follow-up. Ann Oncol 2016;27:v91-v102. [Crossref] [PubMed]
- Meignan M, Barrington S, Itti E, et al. Report on the 4th International Workshop on Positron Emission Tomography in Lymphoma held in Menton, France, 3-5 October 2012. Leuk Lymphoma 2014;55:31-7. [Crossref] [PubMed]
- Martelli M, Ceriani L, Zucca E, et al. [18F]fluorodeoxyglucose positron emission tomography predicts survival after chemoimmunotherapy for primary mediastinal large B-cell lymphoma: results of the International Extranodal Lymphoma Study Group IELSG-26 Study. J Clin Oncol 2014;32:1769-75. [Crossref] [PubMed]
- Juweid ME, Stroobants S, Hoekstra OS, et al. Use of positron emission tomography for response assessment of lymphoma: consensus of the Imaging Subcommittee of International Harmonization Project in Lymphoma. J Clin Oncol 2007;25:571-8. [Crossref] [PubMed]
- Lazarovici J, Terroir M, Arfi-Rouche J, et al. Poor predictive value of positive interim FDG-PET/CT in primary mediastinal large B-cell lymphoma. Eur J Nucl Med Mol Imaging 2017;44:2018-24. [Crossref] [PubMed]
- Cheson BD, Fisher RI, Barrington SF, et al. Recommendations for initial evaluation, staging, and response assessment of Hodgkin and non-Hodgkin lymphoma: the Lugano classification. J Clin Oncol 2014;32:3059-68. [Crossref] [PubMed]
- Ceriani L, Barrington S, Biggi A, et al. Training improves the interobserver agreement of the expert positron emission tomography review panel in primary mediastinal B-cell lymphoma: interim analysis in the ongoing International Extranodal Lymphoma Study Group-37 study. Hematol Oncol 2017;35:548-53. [Crossref] [PubMed]
- Ceriani L, Martelli M, Gospodarowicz MK, et al. Positron Emission Tomography/Computed Tomography Assessment After Immunochemotherapy and Irradiation Using the Lugano Classification Criteria in the IELSG-26 Study of Primary Mediastinal B-Cell Lymphoma. Int J Radiat Oncol Biol Phys 2017;97:42-9. [Crossref] [PubMed]
- Dunleavy K, Wilson WH. Primary mediastinal B-cell lymphoma and mediastinal gray zone lymphoma: do they require a unique therapeutic approach? Blood 2015;125:33-9. [Crossref] [PubMed]
- Vassilakopoulos TP, Papageorgiou SG, Angelopoulou MK, et al. Positron emission tomography after response to rituximab-CHOP in primary mediastinal large B-cell lymphoma: impact on outcomes and radiotherapy strategies. Ann Hematol 2021;100:2279-92. [Crossref] [PubMed]
- Vassilakopoulos TP, Pangalis GA, Chatziioannou S, et al. PET/CT in primary mediastinal large B-cell lymphoma responding to rituximab-CHOP: An analysis of 106 patients regarding prognostic significance and implications for subsequent radiotherapy. Leukemia 2016;30:238-42. [Crossref] [PubMed]
- Zhou H, Xu-Monette ZY, Xiao L, et al. Prognostic factors, therapeutic approaches, and distinct immunobiologic features in patients with primary mediastinal large B-cell lymphoma on long-term follow-up. Blood Cancer J 2020;10:49. [Crossref] [PubMed]
- Le Gouill S, Ghesquières H, Oberic L, et al. Obinutuzumab vs rituximab for advanced DLBCL: a PET-guided and randomized phase 3 study by LYSA. Blood 2021;137:2307-20. [Crossref] [PubMed]
- Hayden AR, Tonseth P, Lee DG, et al. Outcome of primary mediastinal large B-cell lymphoma using R-CHOP: impact of a PET-adapted approach. Blood 2020;136:2803-11. [Crossref] [PubMed]
- Le Gouill S, Casasnovas RO. Interim PET-driven strategy in de novo diffuse large B-cell lymphoma: do we trust the driver? Blood 2017;129:3059-70. [Crossref] [PubMed]
- Casasnovas RO, Meignan M, Berriolo-Riedinger A, et al. SUVmax reduction improves early prognosis value of interim positron emission tomography scans in diffuse large B-cell lymphoma. Blood 2011;118:37-43. [Crossref] [PubMed]
- Vassilakopoulos TP, Michail M, Papageorgiou S, et al. Identification of Very Low-Risk Subgroups of Patients with Primary Mediastinal Large B-Cell Lymphoma Treated with R-CHOP. Oncologist 2021;26:597-609. [Crossref] [PubMed]
- Liu Y, Jiang J, Liu L, et al. Prognostic significance of clinical characteristics and 18Fluorodeoxyglucose-positron emission tomography/computed tomography quantitative parameters in patients with primary mediastinal B-cell lymphoma. J Int Med Res 2022;50:3000605211063027. [Crossref] [PubMed]
- De Mello CA, De Andrade VP, De Lima VC, et al. Prognostic impact of MUM1 expression by immunohistochemistry on primary mediastinal large B-cell lymphoma. Leuk Lymphoma 2011;52:1495-503. [Crossref] [PubMed]
- Ceriani L, Martelli M, Conconi A, et al. Prognostic models for primary mediastinal (thymic) B-cell lymphoma derived from 18-FDG PET/CT quantitative parameters in the International Extranodal Lymphoma Study Group (IELSG) 26 study. Br J Haematol 2017;178:588-91. [Crossref] [PubMed]
- Schmitz N, Zeynalova S, Nickelsen M, et al. CNS International Prognostic Index: A Risk Model for CNS Relapse in Patients With Diffuse Large B-Cell Lymphoma Treated With R-CHOP. J Clin Oncol 2016;34:3150-6. [Crossref] [PubMed]
- Papageorgiou SG, Diamantopoulos P, Levidou G, et al. Isolated central nervous system relapses in primary mediastinal large B-cell lymphoma after CHOP-like chemotherapy with or without Rituximab. Hematol Oncol 2013;31:10-7. [Crossref] [PubMed]
- Récher C, Coiffier B, Haioun C, et al. Intensified chemotherapy with ACVBP plus rituximab versus standard CHOP plus rituximab for the treatment of diffuse large B-cell lymphoma (LNH03-2B): an open-label randomised phase 3 trial. Lancet 2011;378:1858-67. [Crossref] [PubMed]
- Stepanishyna Y, Skrypets T, Novosad O, et al. DA-EPOCH-R VS R-CHOP in Patients with Primary Mediastinal Large B-Cell Lymphoma: Results of Prospective Randomized Ukrainian Multicenter Study. Blood 2020;136:9. [Crossref]
- Xu LM, Fang H, Wang WH, et al. Prognostic significance of rituximab and radiotherapy for patients with primary mediastinal large B-cell lymphoma receiving doxorubicin-containing chemotherapy. Leuk Lymphoma 2013;54:1684-90. [Crossref] [PubMed]
- Giulino-Roth L. How I treat primary mediastinal B-cell lymphoma. Blood 2018;132:782-90. [Crossref] [PubMed]
- Shah NN, Szabo A, Huntington SF, et al. R-CHOP versus dose-adjusted R-EPOCH in frontline management of primary mediastinal B-cell lymphoma: a multi-centre analysis. Br J Haematol 2018;180:534-44. Erratum in: Br J Haematol 2018;181:152. [Crossref] [PubMed]
- Malenda A, Kołkowska-Leśniak A, Puła B, et al. Outcomes of treatment with dose-adjusted EPOCH-R or R-CHOP in primary mediastinal large B-cell lymphoma. Eur J Haematol 2020;104:59-66. [Crossref] [PubMed]
- Wilson WH, Grossbard ML, Pittaluga S, et al. Dose-adjusted EPOCH chemotherapy for untreated large B-cell lymphomas: a pharmacodynamic approach with high efficacy. Blood 2002;99:2685-93. [Crossref] [PubMed]
- Wilson WH, Gutierrez M, O'Connor P, et al. The role of rituximab and chemotherapy in aggressive B-cell lymphoma: a preliminary report of dose-adjusted EPOCH-R. Semin Oncol 2002;29:41-7. [Crossref]
- Held G, Thurner L, Poeschel V, et al. Role of radiotherapy and dose-densification of R-CHOP in primary mediastinal B-cell lymphoma: A subgroup analysis of the unfolder trial of the German Lymphoma Alliance (GLA). J Clin Oncol 2020;38:8041. [Crossref]
- Romejko-Jarosinska J, Ostrowska B, Dabrowska-Iwanicka A, et al. High efficacy of intensive immunochemotherapy for primary mediastinal B-cell lymphoma with prolonged follow up. Sci Rep 2022;12:10551. [Crossref] [PubMed]
- Cairoli R, Grillo G, Tedeschi A, et al. Efficacy of an early intensification treatment integrating chemotherapy, autologous stem cell transplantation and radiotherapy for poor risk primary mediastinal large B cell lymphoma with sclerosis. Bone Marrow Transplant 2002;29:473-7. [Crossref] [PubMed]
- Hamlin PA, Portlock CS, Straus DJ, et al. Primary mediastinal large B-cell lymphoma: optimal therapy and prognostic factor analysis in 141 consecutive patients treated at Memorial Sloan Kettering from 1980 to 1999. Br J Haematol 2005;130:691-9. [Crossref] [PubMed]
- Liu X, Deng T, Guo X, et al. A retrospective analysis of outcomes for primary mediastinal large B-cell lymphoma treated with RCHOP followed by radiotherapy or front-line autologous stem cell transplantation. Hematology 2017;22:258-64. [Crossref] [PubMed]
- Avivi I, Boumendil A, Finel H, et al. Autologous stem cell transplantation for primary mediastinal B-cell lymphoma: long-term outcome and role of post-transplant radiotherapy. A report of the European Society for Blood and Marrow Transplantation. Bone Marrow Transplant 2018;53:1001-9. [Crossref] [PubMed]
- Svoboda J, Bair SM, Landsburg DJ, et al. Brentuximab vedotin in combination with rituximab, cyclophosphamide, doxorubicin, and prednisone as frontline treatment for patients with CD30-positive B-cell lymphomas. Haematologica 2021;106:1705-13. [Crossref] [PubMed]
- Trneny M, Polgarova K, Janikova A, et al. Is it radiotherapy necessary for primary mediastinal B-cell lymphoma (PMBL) patients achieving PET negativity after immunochemotherapy? Hematol Oncol. 2019;37:267-8. [Crossref]
- Castellino SM, Geiger AM, Mertens AC, et al. Morbidity and mortality in long-term survivors of Hodgkin lymphoma: a report from the Childhood Cancer Survivor Study. Blood 2011;117:1806-16. [Crossref] [PubMed]
- Mazonakis M, Lyraraki E, Damilakis J. Second cancer risk assessments after involved-site radiotherapy for mediastinal Hodgkin lymphoma. Med Phys 2017;44:3866-74. [Crossref] [PubMed]
- Xu LM, Li YX, Fang H, et al. Dosimetric evaluation and treatment outcome of intensity modulated radiation therapy after doxorubicin-based chemotherapy for primary mediastinal large B-cell lymphoma. Int J Radiat Oncol Biol Phys 2013;85:1289-95. [Crossref] [PubMed]
- Cwynarski K, Marzolini MAV, Barrington SF, et al. The management of primary mediastinal B-cell lymphoma: a British Society for Haematology Good Practice Paper. Br J Haematol 2019;185:402-9. [Crossref] [PubMed]
- Pinnix CC, Dabaja B, Ahmed MA, et al. Single-institution experience in the treatment of primary mediastinal B cell lymphoma treated with immunochemotherapy in the setting of response assessment by 18fluorodeoxyglucose positron emission tomography. Int J Radiat Oncol Biol Phys 2015;92:113-21. [Crossref] [PubMed]
- Tai WM, Quah D, Yap SP, et al. Primary mediastinal large B-cell lymphoma: optimal therapy and prognostic factors in 41 consecutive Asian patients. Leuk Lymphoma 2011;52:604-12. [Crossref] [PubMed]
- Chan EHL, Koh LP, Lee J, et al. Real world experience of R-CHOP with or without consolidative radiotherapy vs DA-EPOCH-R in the first-line treatment of primary mediastinal B-cell lymphoma. Cancer Med 2019;8:4626-32. [Crossref] [PubMed]
- Goldschmidt N, Kleinstern G, Orevi M, et al. Favorable outcome of primary mediastinal large B-cell lymphoma patients treated with sequential RCHOP-RICE regimen without radiotherapy. Cancer Chemother Pharmacol 2016;77:1053-60. [Crossref] [PubMed]
- Messmer M, Tsai HL, Varadhan R, et al. R-CHOP without radiation in frontline management of primary mediastinal B-cell lymphoma. Leuk Lymphoma 2019;60:1261-5. [Crossref] [PubMed]
- Gagliardi G, Constine LS, Moiseenko V, et al. Radiation dose-volume effects in the heart. Int J Radiat Oncol Biol Phys 2010;76:S77-85. [Crossref] [PubMed]
- van Leeuwen FE, Klokman WJ, Hagenbeek A, et al. Second cancer risk following Hodgkin's disease: a 20-year follow-up study. J Clin Oncol 1994;12:312-25. [Crossref] [PubMed]
- De Bruin ML, Sparidans J, van't Veer MB, et al. Breast cancer risk in female survivors of Hodgkin's lymphoma: lower risk after smaller radiation volumes. J Clin Oncol 2009;27:4239-46. [Crossref] [PubMed]
- Nielsen KM, Offersen BV, Nielsen HM, et al. Short and long term radiation induced cardiovascular disease in patients with cancer. Clin Cardiol 2017;40:255-61. [Crossref] [PubMed]
- Hawkes EA. Can PET eradicate irradiation in PMBCL? Blood 2020;136:2725-6. [Crossref] [PubMed]
- Hamlin PA, Dickson M, Kewalramani T, et al. Relapsed and Refractory Primary Mediastinal Diffuse Large B-Cell Lymphoma: Outcome with ICE-Based Treatment. Blood 2006;108:3057. [Crossref]
- Kuruvilla J, Pintilie M, Tsang R, et al. Salvage chemotherapy and autologous stem cell transplantation are inferior for relapsed or refractory primary mediastinal large B-cell lymphoma compared with diffuse large B-cell lymphoma. Leuk Lymphoma 2008;49:1329-36. [Crossref] [PubMed]
- Aoki T, Shimada K, Suzuki R, et al. High-dose chemotherapy followed by autologous stem cell transplantation for relapsed/refractory primary mediastinal large B-cell lymphoma. Blood Cancer J 2015;5:e372. [Crossref] [PubMed]
- Tao Y, Chen H, Liu D, et al. Survival among patients with composite and sequential lymphoma between primary mediastinal lymphoma/diffuse large B-cell lymphoma and classical Hodgkin lymphoma: A population-based study. Leuk Res 2021;111:106669. [Crossref] [PubMed]
- Sarkozy C, Hung SS, Chavez EA, et al. Mutational landscape of gray zone lymphoma. Blood 2021;137:1765-76. [Crossref] [PubMed]
- Mei Q, Zhang W, Liu Y, et al. Camrelizumab Plus Gemcitabine, Vinorelbine, and Pegylated Liposomal Doxorubicin in Relapsed/Refractory Primary Mediastinal B-Cell Lymphoma: A Single-Arm, Open-Label, Phase II Trial. Clin Cancer Res 2020;26:4521-30. [Crossref] [PubMed]
- Armand P, Rodig S, Melnichenko V, et al. Pembrolizumab in Relapsed or Refractory Primary Mediastinal Large B-Cell Lymphoma. J Clin Oncol 2019;37:3291-9. [Crossref] [PubMed]
- Zinzani PL, Santoro A, Gritti G, et al. Nivolumab Combined With Brentuximab Vedotin for Relapsed/Refractory Primary Mediastinal Large B-Cell Lymphoma: Efficacy and Safety From the Phase II CheckMate 436 Study. J Clin Oncol 2019;37:3081-9. [Crossref] [PubMed]
- Herrera AF, Chen L, Khajavian S, et al. Allogeneic Stem Cell Transplantation Provides Durable Remission in Patients with Primary Mediastinal Large B Cell Lymphoma. Biol Blood Marrow Transplant 2019;25:2383-7. [Crossref] [PubMed]
- Crombie JL, Nastoupil LJ, Redd R, et al. Real-world outcomes of axicabtagene ciloleucel in adult patients with primary mediastinal B-cell lymphoma. Blood Adv 2021;5:3563-7. [Crossref] [PubMed]
- Lazzarino M, Orlandi E, Paulli M, et al. Treatment outcome and prognostic factors for primary mediastinal (thymic) B-cell lymphoma: a multicenter study of 106 patients. J Clin Oncol 1997;15:1646-53. [Crossref] [PubMed]
- Sehn LH, Antin JH, Shulman LN, et al. Primary diffuse large B-cell lymphoma of the mediastinum: outcome following high-dose chemotherapy and autologous hematopoietic cell transplantation. Blood 1998;91:717-23. [Crossref] [PubMed]
- Zinzani PL, Melnichenko V, Bouabdallah K, et al. Pembrolizumab Monotherapy in Relapsed or Refractory Primary Mediastinal Large B-Cell Lymphoma (PMBCL): 3-Year Follow-up of the Keynote-170 Study. Blood 2020;136:42-43. [Crossref]
- Herrera AF, Moskowitz AJ, Bartlett NL, et al. Interim results of brentuximab vedotin in combination with nivolumab in patients with relapsed or refractory Hodgkin lymphoma. Blood 2018;131:1183-94. [Crossref] [PubMed]
- Zinzani PL, Pellegrini C, Chiappella A, et al. Brentuximab vedotin in relapsed primary mediastinal large B-cell lymphoma: results from a phase 2 clinical trial. Blood 2017;129:2328-30. [Crossref] [PubMed]
- Cao AT, Law CL, Gardai SJ, et al. Abstract 5588: Brentuximab vedotin-driven immunogenic cell death enhances antitumor immune responses, and is potentiated by PD1 inhibition in vivo. Cancer Res 2017;77:5588. [Crossref]
- Heiser RA, Grogan BM, Manlove LS, et al. Abstract 1789: CD30+T regulatory cells, but not CD30+CD8 T cells, are impaired following brentuximab vedotin treatment in vitro and in vivo. Cancer Res 2018;78:1789. [Crossref]
- Chiappella A, Dodero A, Guidetti A, et al. Checkpoint Inhibition before Axicabtagene Ciloleucel Cell Therapy in Primary Mediastinal B-Cell Lymphoma (PMBCL) Treated in Real Life Setting. Blood 2020;136:12-3. [Crossref]
- Neelapu SS, Locke FL, Bartlett NL, et al. Axicabtagene Ciloleucel CAR T-Cell Therapy in Refractory Large B-Cell Lymphoma. N Engl J Med 2017;377:2531-44. [Crossref] [PubMed]
- Schuster SJ, Bishop MR, Tam CS, et al. Tisagenlecleucel in Adult Relapsed or Refractory Diffuse Large B-Cell Lymphoma. N Engl J Med 2019;380:45-56. [Crossref] [PubMed]
- Abramson JS, Palomba ML, Gordon LI, et al. Lisocabtagene maraleucel for patients with relapsed or refractory large B-cell lymphomas (TRANSCEND NHL 001): a multicentre seamless design study. Lancet 2020;396:839-52. [Crossref] [PubMed]
- Cappell KM, Sherry RM, Yang JC, et al. Long-Term Follow-Up of Anti-CD19 Chimeric Antigen Receptor T-Cell Therapy. J Clin Oncol 2020;38:3805-15. [Crossref] [PubMed]
- Fenske TS, Ahn KW, Graff TM, et al. Allogeneic transplantation provides durable remission in a subset of DLBCL patients relapsing after autologous transplantation. Br J Haematol 2016;174:235-48. [Crossref] [PubMed]
- Bacher U, Klyuchnikov E, Le-Rademacher J, et al. Conditioning regimens for allotransplants for diffuse large B-cell lymphoma: myeloablative or reduced intensity? Blood 2012;120:4256-62. [Crossref] [PubMed]
- Glass B, Hasenkamp J, Wulf G, et al. Rituximab after lymphoma-directed conditioning and allogeneic stem-cell transplantation for relapsed and refractory aggressive non-Hodgkin lymphoma (DSHNHL R3): an open-label, randomised, phase 2 trial. Lancet Oncol 2014;15:757-66. [Crossref] [PubMed]
- Le Bourgeois A, Labopin M, Blaise D, et al. Reduced-intensity versus reduced-toxicity myeloablative fludarabine/busulfan-based conditioning regimens for allografted non-Hodgkin lymphoma adult patients: a retrospective study on behalf of the Société Francophone de Greffe de Moelle et de Thérapie Cellulaire. Ann Oncol 2017;28:2191-8. [Crossref] [PubMed]
- Le Calvez B, Tessoulin B, Renaud L, et al. Outcomes of Allogeneic Hematopoietic Cell Transplantation (allo-HCT) for Adults with Primary Mediastinal B Cells Lymphoma (PMBCL): A Retrospective Study on Behalf of the Société Francophone De Greffe De Moelle Et De Thérapie Cellulaire (SFGM-TC) and the Lymphoma Study Association (LYSA) Group. Blood 2021;138:1826. [Crossref]
- Camus V, Viennot M, Lequesne J, et al. Targeted genotyping of circulating tumor DNA for classical Hodgkin lymphoma monitoring: a prospective study. Haematologica 2021;106:154-62. [Crossref] [PubMed]
- Pirosa MC, Bruscaggin A, Terzi di Bergamo L, et al. Circulating tumor DNA is a prognostic biomarker at baseline and improves the accuracy of interim PET in classic Hodgkin lymphoma. EHA 2022.
- Bohers E, Viailly PJ, Becker S, et al. Non-invasive monitoring of diffuse large B-cell lymphoma by cell-free DNA high-throughput targeted sequencing: analysis of a prospective cohort. Blood Cancer J 2018;8:74. [Crossref] [PubMed]
- Rossi D, Diop F, Spaccarotella E, et al. Diffuse large B-cell lymphoma genotyping on the liquid biopsy. Blood 2017;129:1947-57. [Crossref] [PubMed]
- Pang D, Jiang X, Huang L, et al. Ctdna Monitoring in Predicting Relapse of Primary Mediastinal B-Cell Lymphoma. Blood 2020;136:36. [Crossref]
- Kurtz DM, Scherer F, Jin MC, et al. Circulating Tumor DNA Measurements As Early Outcome Predictors in Diffuse Large B-Cell Lymphoma. J Clin Oncol 2018;36:2845-53. [Crossref] [PubMed]
- Sarkozy C, Chong L, Takata K, et al. Gene expression profiling of gray zone lymphoma. Blood Adv 2020;4:2523-35. [Crossref] [PubMed]
- Cader FZ, Hu X, Goh WL, et al. A peripheral immune signature of responsiveness to PD-1 blockade in patients with classical Hodgkin lymphoma. Nat Med 2020;26:1468-79. [Crossref] [PubMed]
Cite this article as: Camus V, Drieux F, Jardin F. State of the art in the diagnosis, biology and treatment of primary mediastinal B-cell lymphoma: a review. Ann Lymphoma 2022;6:13.